ENVIRONMENTALLY SOUND
SMALL-SCALE
ENERGY PROJECTS
GUIDELINES FOR PLANNING
by
Elizabeth Ann Bassan
Timothy S. Wood, Ph. D.
Technical Editor
Coordination in Development (CODEL)
Volunteers in Technical Assistance (VITA)
VITA
1600 Wilson Boulevard, Suite 500
Arlington, Virgnia 22209 USA
Tel:
703/276-1800 . Fax: 703/243-1865
Internet: pr-info@vita.org
CODEL
Environment and Development Program
79 Madison Avenue
New York, New York 10016
Illustrations by Linda Jacobs
Cover Design by Susann Foster Brown
CODEL/VITA 1985
ISBN No. 0-86619-171-2
TABLE OF CONTENTS
Preface
Chapter I
USES AND USERS OF
THIS MANUAL
What is the
purpose of this manual?
What does the
manual provide?
Who should use
this manual?
Chapter II
ECOLOGY FOR
SUSTAINABLE ENERGY DEVELOPMENT
What are
ecosystems and biological communities?
How does an
ecosystem work?
Producers
Consumers
Decomposers
Non-living
environment
How are energy and
the environment related?
What is energy
flow?
What is a nutrient
cycle?
What is the
hydrologic (water) cycle?
What are limiting
factors?
What is
renewability?
Energy, ecology,
and the tropics
What are
environmental effects?
Chapter III
SOCIOECONOMIC
CONSIDERATIONS OF ENERGY USE
Energy use in
developing countries
Reaching
participant groups
Social, cultural,
and economic aspects of energy
What is the role
of women in energy production?
Energy and general
welfare
Factors affecting
the adoption of energy technologies
Who pays for
environmental problems?
Chapter IV
ENERGY PLANNING
FOR SUSTAINABLE DEVELOPMENT
Why plan?
Special
considerations in meeting energy needs
What is end use?
How efficiently is
energy used?
Measuring energy
output
Wind
Solar
Water
Forests and
vegetation
Crop residues
Animal residues
Chapter V
ULIPUR,
BANGLADESH: A CASE STUDY
How were
socioeconomic data collected?
How were data
collected on energy use?
How to use the
energy flow diagram
Summing up
Chapter VI
A PROCESS FOR
PLANNING ENERGY PROJECTS
Community
participation
Environmental and
socioeconomic guidelines
Steps in the
planning process
1
Collect information
Community
profile--socioeconomic
characteristics
Natural
resources--ecological
characteristics
Energy use
patterns
2
Identify energy needs and constraints
3
Define project objectives
4
Develop alternative designs
5
Compare alternatives and select one
alternative
6
Implement project
7
Monitor project
8
Evaluate project
Chapter VII
ENERGY SOURCES AND
ENVIRONMENTAL
CONSIDERATIONS
Solar energy
Drying
Cooking
Electricity
generation
Solar ponds
Wind
Water (Hydropower)
Biomass - Fuelwood
- Biogas
- Ethanol
Animal traction
Chapter VIII
MATCHING ENERGY SOURCES
WITH ENERGY USES
Household energy
Cooking
Heating
Lighting
Food processing
Energy for
agriculture
Irrigation
Land
preparation, crop management,
and
harvesting
Chapter IX
SUMMARY
Appendixes
A. ENERGY
CONVERSION TABLE
B. ECOLOGICAL MINI
GUIDELINES
C. TROPICAL
CLIMATES
D. BIBLIOGRAPHY
E. SOURCES OF
GUIDELINES FOR PLANNING SERIES
Environmentally Sound Small-Scale Agricultural Projects,
1979
(Also in Spanish and French)
Environmentally Sound Small-Scale Water Projects, 1981
(Also in Spanish)
Environmentally Sound Small-Scale Forestry Projects, 1983
(Translations in Spanish and French in process)
Can be ordered from:
VITA
Publications
1815 North Lynn Street, Suite 200
Arlington,
Virginia 22209 USA
Preface
This manual is the
fourth volume of the Guidelines for
Planning Series. The series was originally suggested by
representatives of private development agencies to provide
paratechnical information for their field staff and
counterpart
personnel in Third World countries for use in planning
environmentally sound small-scale projects. Titles of other
volumes in the series are listed on the opposite page.
The CODEL
Environment and Development Committee has
guided the development of the Guidelines for Planning
Series.
CODEL acknowledges the contribution of the Committee to this
volume. Those members who reviewed drafts of the manual are
indicated by an asterisk.
Sr. Jean Marie
O'Meara, S.H.C.J., Chairperson
* Ms. Elizabeth
Enloe, Church World Service
Mr. George
Gerardi, Hermandad
* Rev. John L.
Ostdiek, O.F.M., Franciscan Missionary
Union of
Chicago
Mr. Ragnar Overby,
The World Bank
Ms. Agnes Pall,
International Division, YMCA(**)
Mr. C. Anthony
Pryor, U.S. Agency for International
Development
* Sr. Renee Roach,
Medical Mission Sisters
Mr. A. Keith
Smiley, Mohonk Consultations on the
Earth's Ecosystem
In addition, a
number of reviewers offered substantive
comments, which assisted with the preparation of the final
copy:
Mr. Thomas
Carouso, Partnership for Productivity
Ing. Guillermo
Duarte-Monroy, Sistemas
Agroenergeticos
Integrados
Mr. Gary
Eilerts, formerly Appropriate Technology
International
(**) Deceased August, 1983
Dr. Peter
Ffolliott, University of Arizona
Mr. Jack Fritz,
National Academy of Sciences
Dr. Gary
Garriott, Volunteers in Technical Assistance
Ms. Marilyn
Hoskins, Virginia Polytechnic Institute
and State
University
Dr. Clarence
Kooi, US Agency for International
Development/West Africa
Sr. Caroline
Mbonu, Handmaids of the Holy Child
Mr. Mark Ward, Africa Bureau, US Agency for
International
Development (AID)
Margaret Crouch,
VITA publications office, has served as
liaison with CODEL and technical adviser to CODEL for
several
of the volumes in the series. CODEL takes this opportunity
to
thank Ms. Crouch for her past assistance and special
contributions
to this volume.
Ms. Molly Kux, AID
Office of Forestry, Environment, and
Natural Resources, has encouraged and supported the
preparation
of each of the volumes in the series. She has played an
important
role in assisting with identifying authors and reviewers and
personally
reviewing the books. Mr. Albert Printz, AID Environmental
Coordinator, reviewed and commented on the text.
CODEL acknowledges with thanks the continued support and
encouragement for the Environment and Development Program
from both Ms. Kux and Mr. Printz.
The AID Office of
Private and Voluntary Cooperation has
supported the development of the CODEL Environment and
Development Program. CODEL gratefully acknowledges the
contribution of that office and the support of Mr. Paul
Bisek,
Project Officer, for the Program as a whole.
CODEL is pleased to
publish this booklet written by Elizabeth
Bessan in collaboration with Dr. Timothy Wood, Technical
Editor.
During the preparation of this volume Ms. Bassan served with
the
Sierra Club International Earth Care Center and subsequently
with the American Council of Voluntary Agencies in Foreign
Service. Dr. Timothy Wood recently spent two years in West
Africa as a consultant for VITA, returning to his former
position
as Director of Environmental Studies, Wright State
University,
Dayton, Ohio. Brief biographies of the author and technical
editor can be found at the end of the book.
Ms. Wynta Boynes,
American Council of Voluntary Agencies
in Foreign Service, did an excellent job of editing the
text.
Finally, CODEL
acknowledges with thanks the cooperative
services of Ms. Rosa Marsala, Ms. Gwen Dantzler, and Ms.
Betty
Wynn of the Unified Information System, of the Support
Agency
of the Presbyterian Church (U.S.A.).
We welcome comments
from readers of the book. A questionnaire
is enclosed for your convenience. Please share your
reactions with us.
Rev. Boyd
Lowry, Executive Director, CODEL
Ms. Helen L. Vukasin and Sr. Mary Ann
Smith
Environment and Development Program, CODEL
ABOUT CODEL
Coordination in Development (CODEL) is a private,
not-for-profit
consortium of 38 development agencies working in developing
countries. CODEL funds community development activities that
are locally initiated and ecumenically implemented. These
activities include health, agriculture, water, appropriate
technology, and training projects, among others.
The Environment and Development Program of CODEL serves the
private and voluntary development community by providing
workshops, information, and materials designed to document
the
urgency, feasibility, and potential of an approach to
small-scale
development that stresses the interdependence of human and
natural resources. This manual is one of several materials
developed under the Program to assist development workers in
taking the physical environment into account during project
planning, implementation, and evaluation. For more
information,
contact CODEL Environment and Development Program at
79 Madison Avenue, New York, New York 10016 USA.
ABOUT VITA
Volunteers in Technical Assistance (VITA) is a private
nonprofit
international development organization. It makes available
to
individuals and groups in developing countries a variety of
information and technical resources aimed at fostering
self-sufficiency:
needs assessment and program development support;
by-mail and on-site consulting services; information systems
training; and management of field projects. VITA promotes
the
use of appropriate small-scale technologies, especially in
the area
of renewable energy. VITA's extensive documentation center
and
worldwide roster of volunteer technical experts enable it to
respond to thousands of technical inquiries each year. It
also
publishes a quarterly newsletter and a variety of technical
manuals and bulletins. For more information, contact VITA at
1815 N. Lynn Street Suite 200, Arlington, Virginia 22209
USA.
Chapter I
USES AND USERS OF THIS MANUAL
What is the purpose of this manual?
The purpose of this
manual is to help development workers
and others to become aware of the environmental factors that
should be considered in planning small-scale energy projects
that
are environmentally sound and therefore more likely to be
sustained.
Environmentally
sound planning includes the physical environmental
factors as well as the socioeconomic and cultural factors.
This approach helps assure the protection of the renewable
natural resources that supply most of the energy used in the
Third
World.
Traditional
sources--dung, crop and forest residues, fuelwood,
and human and animal energy--make up a very significant
amount
of the energy used in developing countries. Estimates of how
much traditional fuels are used vary, largely because of the
difficulty in measuring non-commercial fuel use.
Recent
estimates indicate that in Asia these fuels account for
about 65
percent of total energy use, in Africa, about 85 percent,
and in
Latin America, about 20 percent. This masks the enormous
variation both between and within countries.
It is not likely
that the situation will change dramatically in
the near future. Because of supply and cost factors many
energy
specialists doubt that developing countries will make the
transition to fossil fuels as has occurred in developed
countries.
From an environmental point of view, this may be good. For
development, the challenge is to provide energy essential
for
socioeconomic development, and to promote resource use that
will allow for sustainable, reliable supplies of energy.
Traditional,
renewable fuels have long been considered the
most environmentally sound. Practice has shown that this is
true
if they are not used beyond their ability to replace
themselves.
Environmental damage occurs when "renewable"
resources are
treated as a product that is used faster than it can be
replaced.
This can damage the ecological system, leading to soil
erosion and
degradation, loss of watersheds, increased flooding, and
desertification.
This destroys the ability of the land to produce.
Agricultural productivity and energy availability--that is,
having
food to cook and fuel with which to cook--depend on the
ecological
well-being of the physical environment.
Energy is critical
to development. Energy is necessary for
cooking and for pursuing productive activities that generate
income and provide employment. This is as true of the twigs
and
leaves for village fires as for the relatively small amounts
of
fossil fuels that represent the life-blood of market town
activities.
Energy can improve the quality of life by providing drinking
water, light, and heat. It can be used in devices that lead
directly
to added income, or free up time that can be used for other
purposes.
When planning
projects that involve the use of energy, there
is a tendency to deal with energy and environmental
questions in
isolation and so to ignore their relationships to other
issues. In
examining these questions, planners must consider the
relevant
social and economic factors as well as the technical.
Finally,
they should appraise administrative and/or implementing
capabilities. For regardless of the size of the effort, good
energy
planning requires more than merely a technology, a source of
funds, and sound development intentions. The purpose of this
manual then, is to aid development workers in thinking
through
how to use natural resources for energy in a way that
maintains
ecological well-being--the lifeline for survival.
What does the manual provide?
It provides:
*
an introduction to ecological concepts,
their relevance to
energy
development, and their interaction with the
broader
socioeconomic environment in which energy
development
takes place
*
a guide to planning small-scale energy
projects in which
environmental
costs and benefits are incorporated
*
guidelines for making an informed decision
on the most
enviroamentally
sound energy project alternative
*
an overview of the environmental
considerations in using
various energy
sources
*
background information for choosing an
environmentally
sound strategy
to provide for specific energy end-uses, in
households,
agriculture, small-scale industry, and
transportation
*
a useful reference to commonly used energy
and
environmental
terms
*
a look at alternative solutions to
addressing energy
development within the broader framework
of environmental
and economic
considerations.
Who should use this manual?
This manual was
prepared for development workers and
project planners in Third World countries who are assisting
the
urban and rural poor to plan and implement small-scale
energy
projects. It has been written for those who lack technical
training
in the area of energy, but require some general guidelines
for
planning projects that will help to meet pressing energy
needs and
at the same time protect and even increase the renewable
resources.
Chapter II
ECOLOGY
FOR SUSTAINABLE ENERGY DEVELOPMENT
Ecology is the
study of the relationships among all living
things and their surroundings, or environment.
Generally the
environment is thought to include such things as land,
vegetation,
climate, shelter and animals.
In this manual a concept of human
environment is expanded to include cultural, economic,
social, and
political factors.
This chapter will
introduce a few ecological principles that
are important to the planning of sound energy projects.
A more
detailed treatment of ecological processes can be found in
any
basic ecology text.
What are ecosystems and biological communities?
A central theme
of ecology is the concept of an ecosystem.
Common examples include forests, mangrove swamps,
grasslands,
and oceans. The
plants and animals in an ecosystem form biological
communities. Members
of the communities are like interwoven
threads of a fabric, each performing an important role that
helps the whole community to function.
Some of the "threads"
may be energy and minerals that combine in complex ways to
form a "food web."
Other "threads" may involve activities that
aerate and fertilize the soil, help the soil retain
moisture,
pollinate flowers, and aid in seed dispersal, to name just a
few.
Although no two
ecosystems are identical, all have the same
fundamental structure.
Two basic processes of all ecosystems
are: (1) the one-way
flow of energy, and (2) the cyclical flow of
mineral nutrients.
These processes are strongly influenced by
such physical factors as sunlight, water, and
temperature. Energy
is expended and nutrients are recycled through eating.
Eating
links all plants and animals to each other.
The way in which the
linking takes place is called a "food chain."
How does an ecosystem work?
Ecosystems tend
to be self-regulating. In
well-functioning
ecosystems processes of growth and decomposition occur at a
rate
and in a manner to maintain a balance or equilibrium.
A
development project that introduces a new component to the
ecosystem (for example, waterpower) or diverts resources
useful
to the ecosystem (for example, organic wastes) may change the
balance or equilibrium.
Sometimes a new equilibrium can be
quickly achieved. In
other cases, the ability of the ecosystem to
foster growth is changed.
There are four
"actors" in any ecosystem through which
energy and nutrients flow:
1.
Producers -- green plants such as algae in a
pond, grass in
a field, or
trees and undergrowth in a forest. The
producers
make life possible through their ability to
convert
radiant energy from the sun to chemical energy
using carbon
dioxide and water. The process is
called
photosynthesis. Other living
things, including people, can
use this
energy for food and fuel. About 100
billion tons
of organic
matter are produced annually through
photosynthesis.
Eventually, most of this is changed back
to carbon
dioxide and water. Some is left
temporarily in
vegetation,
and some becomes cell tissue in people and
other
animals.
2.
Consumers--animals (including people) that eat
plants
and/or other
animals. Part of what is eaten becomes
energy stored
in cell tissue. The energy is used for
growth,
movement, reproduction, and the maintenance of
the body
(respiration, digestion, etc.).
3.
Decomposers--bacteria and fungi.
These produce
enzymes that
break down dead plant and animal material.
This releases
essential nutrients, which may be reused by
producers. It may also provide
organic materials that
bind soil
particles and thus help protect the soil from
erosion.
4.
Non-Living Environment--basic elements,
combinations of
elements, and
climate. Basic elements include carbon,
phosphorus,
nitrogen, and sulphur, among others.
Combinations
of elements
include proteins, carbohydrates, and
fats.
The climate, which affects the rate of
growth and
decomposition, includes temperature, moisture, and
sunlight.
As noted, the
components of an ecosystem are complex and
interwoven. Each
performs an essential role that fosters the
growth of the living parts and maintains the entire
system. And
changes in one component will affect not only its own
functions,
but also its relationship with the others--and the
functioning of
the system as a whole.
How are energy and the environment related?
In less
advantaged countries much of the energy consumed is
derived from organic matter, such as crop residues, animal
dung,
trees, and shrubs.
These same materials may also be used for
fertilizer or construction.
They may be needed by plants and
animals for food, nutrients, and shelter.
Such competition for
resources can have a far-reaching impact that may not be
apparent immediately.
A more obvious
environmental impact occurs whenever any
energy resources are exploited and used by man.
Inevitably,
water, air, and soil pollution are the result.
Currently in many
developing countries, for example, wood for charcoal is
being cut
faster than it can be replaced.
Proper management techniques,
such as timely replanting, more efficient charcoal
production
methods, and controlled harvest rates, are not being
adequately
practiced.
Exploiting the wood energy resource can become an
important contributing cause of deforestation.
The results of
deforestation include exposing soils to direct
sunlight, heavy rains, and nutrient loss.
Soils become dry, compacted,
and unproductive.
Soil erosion leads to huge silt deposits
in streams, creating dry stream beds and reducing the
effectiveness
of dams and irrigation channels.
As the wood supply diminishes,
the price of fuelwood increases, either in cash amounts or
in the time and effort required to bring it in from more
distant
areas. Eventually,
people may begin to use alternative fuels such
as cow dung, which precludes its use as an important soil
conditioner and fertilizer.
The purpose of
understanding ecology in relation to development
projects is to project the effect a proposed project may
have on an ecosystem, to learn what mitigating measures may
be
required, and to monitor changes in the ecosystem as the
project
is implemented.
This manual
addresses the conflicts between the uses of
energy and the natural resources that provide energy.
We are as
concerned with the impact energy use may have on the
environment
as with the impact that environmental degradation may
have on the potential energy supply.
Development planners who
can see beyond the technical limitations of energy
technologies to
view the relationship between economic development and
resource management will have developed one additional
method
for assessing project feasibility and promoting economically
viable, sustainable projects.
What is energy flow?
All life depends
on energy to grow and reproduce. The
ultimate source of energy on earth is the sun, which
transmits its
energy in the form of radiation.
Green plants make life possible
because they are able to convert radiant solar energy to a
chemical form, using carbon dioxide and water.
This process is
called photosynthesis.
Chemical energy is passed along as food to
plant-eating animals, such as some insects, birds, rodents,
wild
and domestic animals, and people.
These animals use much of the
energy for their own activities, then transfer the
rest--again as
food--to predators or to decomposing bacteria and
fungi. (See
diagram page 8.)
esed1x8.gif (540x540)
The diagrams on
the following pages show how solar energy is
esed2110.gif (486x486)
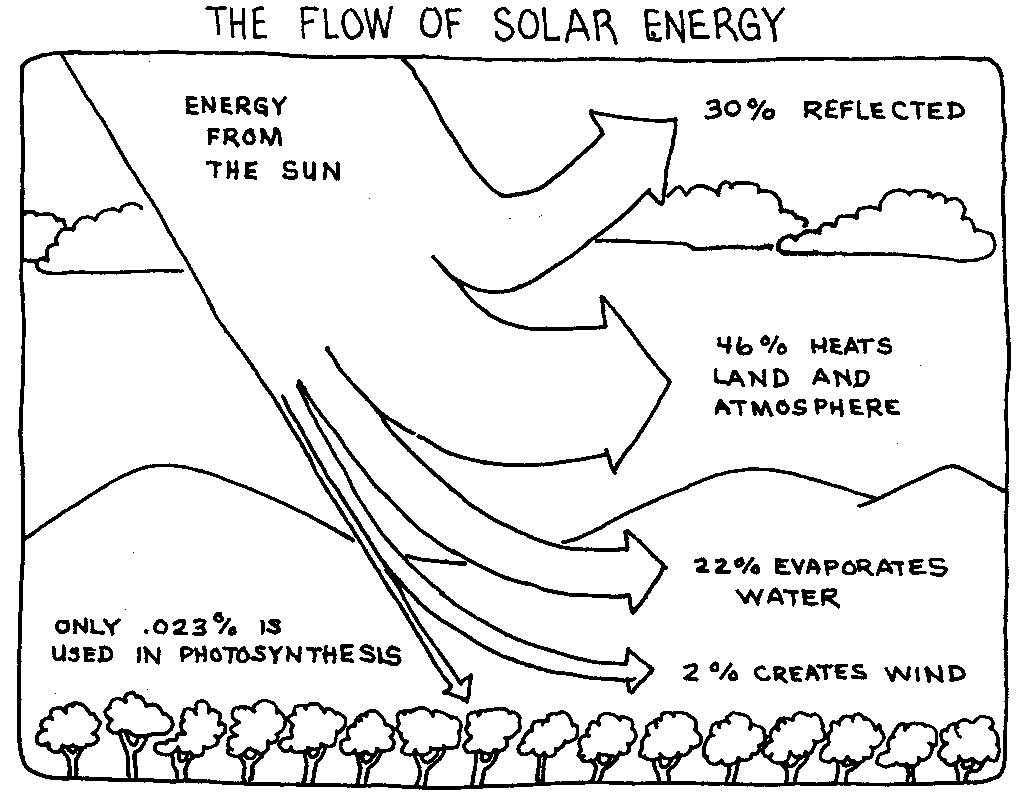
photosynthesis.
Smaller and smaller amounts of energy are passed
along from the plants to others in the food chain.
This is because
a lot of food energy is used in heat-producing activities,
and this
heat energy is dissipated into space.
Only a small amount of the
initial energy is stored in chemical form, which becomes
food in
the food chain.
All forms of
energy undergo the processes of conversion and
dissipation like that just described for solar energy.
These two
characteristics of energy are the first and second laws of
thermodynamics: (1)
energy cannot be created nor destroyed, but
only converted to other forms, and (2) as energy flows
through an
ecosystem, it is degraded and eventually dissipated into
heat, a
non-usable form.
This means that a continuous supply of solar
energy is required to support life.
What is a nutrient cycle?
The cyclic flow
of nutrients involves both living and nonliving
parts of ecosystems.
(See diagram on page 8.) The decomposers
play a major role in recycling nutrients by breaking down
dead plant and animal material.
This makes essential elements
available to the soil and to plants.
Such elements as carbon,
calcium, nitrogen, phosphorus, and sulfur are passed in this
way.
No living thing
can survive without the basic elements.
Removing plant material takes away an important source of
nutrients, and will eventually decrease the fertility of the
soil.
Care must be taken to assure that sufficient ground cover is
left.
Unlike energy,
the minerals essential to life may be used over
and over, constantly recycled within the ecosystem.
In land-based
ecosystems, minerals are taken from the soil by plant roots.
Later they may be passed from plants to grazing animals and
then
to a chain of predators or parasites.
Eventually they are returned
to the soil through the action of decomposers, such as
bacteria
and fungi.
Mineral recycling
is seldom perfect, and in fact can be
seriously disrupted.
For example, wood or cow dung will
eventually decompose if left alone, and the nutrients they
contain
will return to the soil.
When wood or cow dung is collected and
burned for fuel, however, all of the minerals are released
in
smoke or ashes. This
represents a net loss of nutrients from areas
where the fuel was taken, and the soil there may become less
fertile. With the
nutrient cycle thus broken, the ability of the soil
to support plant and animal life is reduced.
What is the hydrologic (water) cycle?
Another important
ecological cycle is the water, or
hydrologic, cycle.
Not only is water necessary for life, it also
helps distribute nutrients.
Powered by solar energy, the
hydrologic cycle is the movement of water from the surface
of
the earth to the atmosphere and back to the earth again.
As can be seen
from the diagram below, forests and other
esed4x13.gif (486x486)

vegetation play very important roles in the hydrologic
cycle.
Vegetation acts to help slow down and control the flow of
water
to an open body of water.
This keeps nutrients within an area,
and prevents flooding and soil erosion.
Land clearing can
significantly affect the process, and may eventually
decrease
agricultural productivity.
What are limiting factors?
To thrive in any
given situation, plants and animals must have
the basic materials for reproduction and growth.
These include
sunlight, water, a wide variety of minerals, shelter, and
protection
from parasites and predators.
When any one of these factors
is present only in amounts approaching the minimum needed
for
survival, it is known as a limiting factor.
For example, the
number of plants and animals that can be
supported in a fertile flood plain is greater than in an
arid upland
of the same area because more water, nutrients, and better
soils
are available.
Nutrients are continually brought into flood plains
from upland regions, and flood plain farmers benefit from
this
transfer of resources. <see image>
esed5x15.gif (486x486)
However, if the
amount of any particular nutrient were
reduced below a critical level, the productivity of the
flood plain
would suffer, even if all other conditions remained the
same. This
nutrient would be the limiting factor.
Limiting factors
will vary from one place to another and
from year to year.
Temperature, the amount and intensity of
rainfall, soil characteristics, sunlight, and availability
of nutrients
vary constantly.
These variations determine the types of species
and number of individual plants and animals that can live in
a
given area.
The amount of
living material an ecosystem produces can be
altered, by natural and human interventions.
Natural causes
include things like violent storms, earthquakes, or drought.
Humans can supplement a limiting material, for example, by
adding water or fertilizer.
However, unintended or unavoidable
human interventions also can decrease the amount an
ecosystem
can produce. For
instance, if plant materials that normally fall to
the ground and decompose to enrich the soil are instead
gathered
for fuel, soil fertility will decline.
Often, biological
potential and productivity can be improved
by adjusting the availability of limiting factors.
For example,
agricultural production can often be increased by adding
whatever
is missing or in limited supply to the area.
This addition might be
fertilizers, organic matter, water, or pest management.
The limiting
factors should be considered in any project in
which energy may be derived from biomass sources that may
have
agricultural uses.
For example, obtaining energy by burning
agricultural residues diverts the amount of nutrients that
can be
returned to the soils.
When nutrients are the limiting factor, this
practice may have serious effects on long-term agricultural
productivity.
However, if these nutrients are not a limiting
factor because agricultural productivity is relatively high
and
there is an excess of agricultural residues, then use of
these
residues for energy can be extremely beneficial to people
who
need fuel.
When considering
limiting factors, remember:
*
Satisfying the most obvious limiting factor
may not solve
the
problem. In fact, satisfying one
limiting factor may
reveal yet
another. For example, when nitrogen is
lacking
in a corn
field, farmers may add a nitrogenous fertilizer.
They may find
that crop growth then is limited by a lack
of phosphorus.
*
Changing existing conditions by altering the
limiting
factors may
upset the relationships among organisms.
Changes in the
system may favor organisms that
previously
were less competitive. These changes
can be
beneficial for
energy production. The degree of
adverse
impact can be
affected by the natural resource
management
planning in the original project design.
What is renewability?
Resources are renewable
if they can reproduce themselves
(for example, plants) or if they are unlimited in supply
(for
example, wind and sunlight).
All plant-based resources have the
ability to reproduce.
However, their reproduction depends on the
presence of suitable soil, sunlight, water, and favorable
temperature.
These limiting factors must be maintained if the resource
is to remain renewable.
An ecosystem can
be degraded in several ways that will
hinder its ability to provide the conditions necessary for
its parts
to reproduce. For
example, dung plays a major role in recycling
nutrients when left on the soil.
When burned as dung cakes for
fuel, many of its nutrients are lost.
Although dung is renewable,
its use as energy may affect its alternative use to improve
soil
fertility. If the
soil is less fertile, less fodder will be produced.
This will eventually also reduce the production of dung.
One way to avoid
this would be to use the dung in biogas
digesters, where sludge by-product can be an excellent
fertilizer.
The availability of other renewable energy sources should
also be
investigated.
Perhaps another energy source would relieve the
need to use as much dung for fuel. <see image>
esed6x18.gif (393x540)
Renewability also
depends on how much time one has for
planning.
For example,
population growth and development pressures
may require that the natural capacity for renewability of
trees be
supplemented with forest management practices.
The time for
planning must be sufficient for forest growth.
Energy, ecology, and the tropics
Ecological
differences occur among tropical arid, tropical
moist, temperate, or other types of climate.
These variations
affect the availability of energy resources and the
environmental
impact of their use.
We cannot cover the range of possible
variations of ecosystems and energy resources in this
manual.
Some of the major regional systems that might affect
tailoring
the discussion in this manual to your particular
surroundings are
examined in Appendix C.
In general, solar
radiation in the tropics is more abundant and
harsh. Rainfall is
usually more variable and concentrated.
Natural rates of soil erosion are higher.
The growth of plants is
faster (except in the more arid areas) and often not
interrupted
seasonally. Differences
[within the tropics are due to: the
quantity
and seasonal variation of rainfall; soil characteristics and
erosion potential; insolation (the rate of delivery of solar
radiation);
and wind patterns.
These characteristics of the tropics as
related to the subject matter of this manual are further
explored
in Appendix C.
What are environmental effects?
Environmental
effects are changes in the environment caused
by human activities or natural processes.
Determining the potential
effects of a particular project requires looking at
economic,
cultural, and social factors, in addition to those factors
that make
up the natural environment.
Some of these factors are explored
in Chapter VI. The
development planner as well as the ecologist
needs to be concerned with determining the amount of
pressure
that populations, communites, and ecosystems can withstand
without being seriously damaged.
Small-scale
energy projects can have both positive and
negative effects.
The impact of any project may be smaller or
much larger than the scope of the project itself.
Changes caused
by a project may not be seen for several years.
It is important to
know current energy use patterns in an area to determine how
development projects may help solve the problems of
acquiring
and using energy. It
is also important to find the relationships
between current energy sources and the natural resource base
of
the project area.
Once those linkages are known the planner must
decide whether:
*
small-scale energy projects will relieve
shortages in local
energy supply
*
the potential source of energy (flowing
streams, fuelwood,
etc.) has
other uses that would compete with energy
production
*
the uses competing with energy production can
be
provided for
in some other way without additional
pressure on
the ecosystem
*
small-scale energy projects in the area will
have negative
environmental
effects
*
environmental damage that may limit energy
supply can
be halted by
developing projects that improve the
management of
natural resources
Charcoal
production provides a useful example of the way
energy production can adversely affect the environment, and
the
way environmental quality can affect energy production.
The
increased use of charcoal, especially in urban areas, is
often one
of the few ways that large numbers of people can cope with
rising
petroleum prices.
In many places
(Haiti, for example), wood for charcoal is
being culled beyond a sustainable point.
Trees are being cut down
faster than they can grow back.
The result is fewer trees, and
therefore less wood for charcoal-making.
At the same time,
deforestation leaves the soil unprotected
from hard rain, leading to nutrient loss and soil
erosion. As the
environmental quality declines, the ability of the ecosystem
to
grow trees at all is undermined, further reducing the wood
available for charcoal-making.
This illustrates
the interrelationship between the effects on
the environment and the balance of the ecosystem as energy
is
produced and used.
The link between
the well-being of people and the availability
of energy is very strong.
This is especially evident in places
where energy is scarce and great efforts or large
proportions of
income are spent to obtain it.
Examination of the relationships
between natural resources, energy, and economics will help
to
find the options for dealing with shortages.
Increasing energy
sources is only one possible solution.
Others may include
institutional changes, improvements in marketing, or the
promotion of soil and water conservation practices.
In all cases,
however, natural resource implications must be realized and
provisions made for careful advance planning and long-term
subsequent monitoring.
Chapter III
SOCIOECONOMIC CONSIDERATIONS OF ENERGY USE
Energy, the
capacity to do work, is the motive force underlying
all activity. Like a tool, it is always used to do something
else--to cook food, light a room, supply power to a piece of
equipment, operate a factory. Like any tool, it has little
value
except when in use.
How energy is
used by people and communities and for what
it is used vary by region, culture, and income group.
Determining
how and for what energy is used, as well as what it could be
used
for, and who controls the sources are critical steps in
energy
planning. In most communities, women can play a central role
in
developing answers to these questions.
There is also a
whole range of variables that need to be
recognized. Some of these are:
-- who
determines access to energy
-- where energy
is produced
-- where energy
is consumed
-- use patterns
to which people presently conform
-- myths
-- demographic
trends
This chapter
will explore some of these issues.
Energy use in developing countries
Developing
countries produce and use energy, especially from
renewable energy resources, in different ways. <see
image> Some villages
esed7x23.gif (437x437)
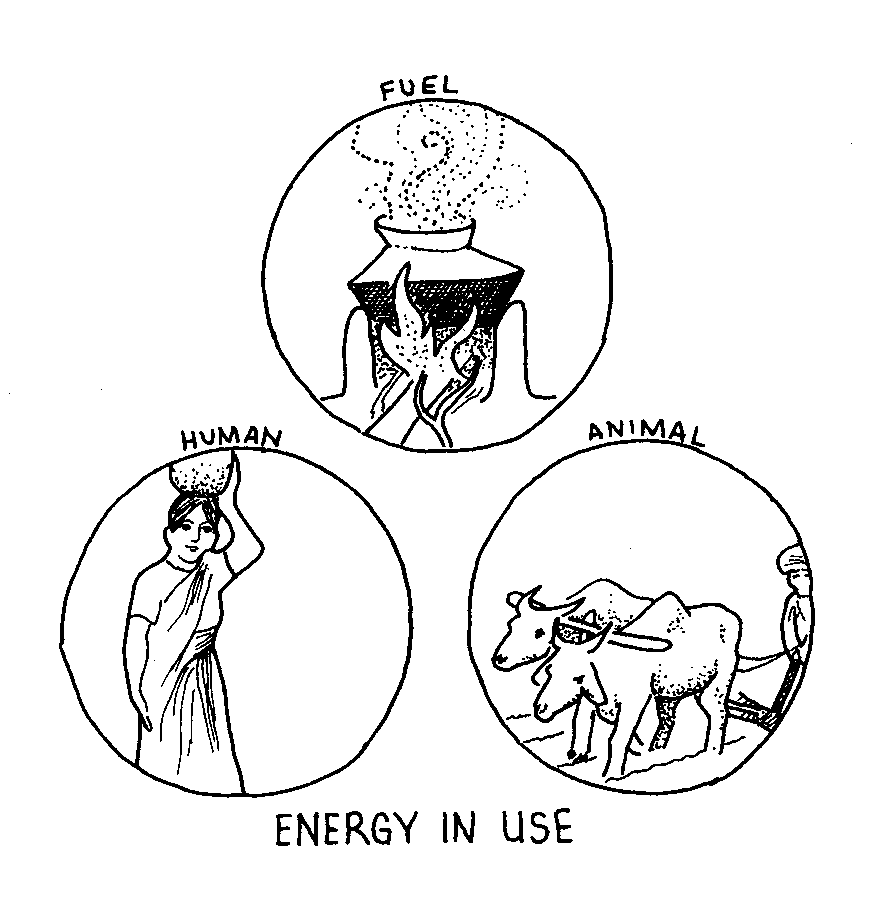
mostly use crop residues. Other villages are more dependent
on
fuelwood. Still other villages depend heavily on dung,
charcoal,
and biogas. This is because the resources that provide
energy are
available in different amounts around the world. Also,
because
the resources that provide energy can be used for a variety
of
other purposes, people may choose differently on the final
use of
a resource.
DAILY ENERGY USE PER CAPITA: AN INDIAN VILLAGE (population,
500)
Activities (Kilocalories/per
capita/day)
Energy
Agriculture Domestic
Lighting
Transport Manufacturing
Total
Sources
(mostly
cooking)
Human Labor
370 250
--
50 10
670
Animal Power
840 0
0
160 0
1000
Fuelwood, Dung
0 4220
0
0 470
4690
Agric. Wastes
___ ____
___
___ ___
____
Total
Non-Commercial
1210 4470
0
210 480
6360
Oil
50 0
260
0 0
310
Coal
0 90
0
0
0
90
Electricity
90 0
40
0 0
130
___ ___
___
___ ___
____
Total
Commercial Energy
140 90
300
0 0
530
Total
1350 4560
300
210 480
6890
Percentage that is
Non-commercial
89% 98%
0%
100% 100%
92%
Source: Holland, et al (1980).
In general,
however, energy use in developing countries,
especially in rural areas, relies heavily on `traditional'
sources--human and animal energy, fuelwood and wood scraps,
charcoal, dung, and crop residues. This is true for about
200
million people.
The table opposite
shows energy use in an Indian village,
which is one example of how energy is used in the developing
world. In this village, human labor, animal power, fuelwood,
dung,
and agricultural wastes provide 92 percent of the energy.
The
bulk of this, or 70 percent, is for cooking.
Reaching participant groups
The table
opposite does not show how energy is distributed
among income groups. Since energy projects affect particular
groups in different ways, this is important information for
helping
the group with which you are working--the participant
group--and
for ensuring that other groups are not adversely affected.
This
information will also help avoid environmental damage, since
groups that are hurt might be forced to collect energy
sources
beyond a sustainable point.
For example, when
biogas digesters were widely distributed
in India, middle-income groups benefited, but poorer groups
were
often worse off than before. The poorer families did not own
enough cattle to produce the dung necessary for the
digesters.
These same families had relied on free dung for fuel. When
the
biogas digesters were introduced, dung suddenly became
valuable
and could no longer be collected for free. This forced the
poor to
find other energy sources or to reduce their energy
consumption.
Finding other sources of energy often resulted in the over
utilization of resources. Making do with less energy can
also
result in nutritional and health problems.
Information on
the use of energy by families and income
groups was collected in a study of the village of Ulipur, in
Bangladesh (Briscoe, 1979). This study (discussed in detail
in
Chapter V) illustrates that when the socioeconomic structure
of a
community leaves some families in poverty, those families
may
create environmental problems in the struggle to survive.
Social, cultural, and economic aspects of energy
The way energy is
obtained and used in households, for
farming, and in small-scale industries is related to social,
cultural, and economic considerations. There is often an
imbalance among these considerations.
For instance, the use of
human waste in biogas digesters often depends more on
cultural
traditions, social organization, and living patterns than
economic
considerations. There may be taboos against using human
wastes,
in which case a central latrine or carting human wastes to a
central point may be unacceptable.
Cultural habits
affecting the use of energy are sometimes
related to environmental factors. In some places, especially
hot,
humid areas, smoke from indoor cookstoves is perceived to be
a
good thing because it discourages harmful insects. In such
areas,
stoves with chimneys should be coupled with housing
improvements
or stove adaptations to keep insects away. In other places,
especially in highland areas, where respiratory ailments and
eye
inflammation from smoke are more of a problem than insects,
stoves with chimneys can be an improvement.
The value of a
new technology is often closely related to its
ability to suit or adapt to sociocultural habits. For
instance,
Lorena (sand and clay) stoves in some parts of Honduras were
altered to suit particular cooking styles and fuelwood
lengths to
the point of sacrificing energy efficiency. (NAS, 1982) The
economic and environmental benefits--using less wood--were
less
important to the users than maintaining customary cooking
styles. Apparently, even the most efficient wood-burning
stoves
can be rejected (and environmentally problematic) if they
cannot
be adapted to local cooking practices.
Similarly,
social, cultural, and economic factors may help
explain the differences in experience with biogas in China
and
India. The rural Chinese are more willing to sacrifice
private gain
for the good of the community. Technology is viewed as
useful
when it serves community needs. Consequently, biogas
digesters
are widely used in a collective way, thereby benefiting the
whole
community.
In contrast,
where a rigid caste system still exists in parts of
India, and energy-producing resources are controlled by a
small
group, individuals work for private gain. Technical
solutions are
sometimes given high priority even when benefits are
marginal
In this case, biogas digesters are acquired by a few wealthy
families for private use partly for the satisfaction of
using
something new and "modern."
The social,
cultural, and economic factors create boundaries
within which energy technologies and projects must be
developed.
For example, stoves need to be adapted to the requirements
of
cooking styles (e.g., frying, simmering, and boiling), and
to
accommodate to the kind of fuel used. Where new charcoal
stoves were introduced in Upper Volta, women used these
stoves
for cooking small amounts of food quickly, but for large
amounts
of slow-cooked foods the women continued to use the
traditional
three-stone fire (NAS, 1982). This may have been to obtain
the
right kind of heat, or because charcoal is too expensive for
extended usage. Both considerations would be important in
designing an acceptable energy project.
What is the role of women in energy production?
Women are key
people in the collection and use of energy in
developing countries. However, this does not mean that women
are therefore in control of the sources of energy. Up to 85
percent of non-commercial energy sources in developing
countries
are used in households for cooking, heating, and lighting.
It is the
women who usually fetch water, collect wood, prepare grains
and
vegetables, make the fire, and cook the food.
Recent surveys
have been made of the expenditure of energy
by men as compared with women in various parts of the world.
The following table indicates that in subsistence economies
women work longer hours than men, and, in most cases, spend
a
substantial amount of their working hours on processing and
preparation of food, gathering fuel, and carrying water.
Introducing energy projects to reduce the use of human
energy in
these activities will have a substantial impact but will
affect men
and women differently.
The greater
contribution of women to survival tasks that
draw directly on local resources means that women have a
special
understanding of the extent, potential, and changes in the
natural
resources in their area. Other activities that bring women
into
constant contact with their environment include raising
vegetables and fruit in home gardens, raising small animals
that
graze nearby, assisting in home construction, preparing a
variety
of medicines, as well as fashioning tools, handicrafts,
cloth, and
dyes from local vegetation and other local materials.
Where
fertile land has been replaced by deserts or where soil has
been
degraded in both semi-arid and humid regions, there is a
serious
shortage of resources to sustain these activities.
TIME
SPENT ON RURAL ACTIVITIES BY WOMEN AND MEN
Country Average
hours of Food for human
Firewood
Water
work/day (in
hrs.) consumption
Female
Male
Female Male
Female Male
Female Male
(in hours)
(in hrs)
(in hrs) (in minutes) (in
minutes) (in minutes)
JAVA 11.1
8.7
2.7 hrs. 6.26
min. 5.25
12.5 -
-
NEPAL 10.8
7.5
3.0 27
minutes 22.8
14.4 40.2
4.2
hours
UPPER
VOLTA 9.8
7.55
2.2 10.0
6.0
2.0 38.0
-
hours
minutes
INDIA 9.69
5.68
3.65 18.0
39
34 74
2.4
hours
minutes
Source: Tinker (1982) based on time-budget studies by
following: Java: White, 1976; Nepal:
Achrya and
Bennett, 1981; Upper Volta: McSweeney, 1980; India: A. K. Reddy, 1980.
Tinker
provides additional data based on fuelwood-gathering studies, which frequently
indicate
longer times due to shorter period, seasonality, and other factors.
South
India - daily - men .72, women .84, children .6 = 2.16 hours/day
Tanzania - weekly - 12 hours on the average
Kenya
- daily - 1/2 - 1 hour/day
Tinker has
observed the critical fuel crisis is in urban not rural areas.
A social forester
described the situation in a Senegalese
village where much of the surrounding wood was cleared for
raising groundnuts, a cash crop (Hoskins, 1979). The
distance and
time required to collect wood increased. More people were
drawn
into the activity to decrease the number of trips and leave
enough
time for all the other household work. Women began to use
other
fuels and more crop residues. They used green wood even
though
they knew it gave less heat and would damage the forest
resource
over time. They began to use dung even though they were
aware
that it was needed to fertilize their gardens. They were
also
forced to purchase wood.
Clearing land for
cash crops forced a change in fuel sources
and uses that affected many aspects of daily living in the
Senegalese village.
Less fuel and more time spent gathering
meant that the quality and quantity of food changed. To save
fuel, women switched from cooking two hot meals a day to one
a
day, or one every other day. They also turned to
rapidly-cooked
foods and to serving raw food. Fewer vegetables were served
because the women had less time to tend their gardens and
also
found that their gardens grew less food--they complained of
loss
of ground cover that had provided natural fertilizers.
Buying
fuelwood left less money to buy food. Changes in diet affect
health and nutrition, which affect the life-long productivity
of
people.
When developing
energy projects, it is important that women
participate from beginning to end. Their unique knowledge of
the
available natural resources is essential to a good project.
Only
they truly know their needs, the time they can devote to a
project, whether it will benefit them (an absolute necessity
for
continued participation), and whether project ideas are
compatible with environmental, social, cultural, and
ownership
conditions in their community.
Energy and general welfare
Fuel availability
affects many other facets of life, such as
education and employment. Children may be kept home from
school because mothers cannot both travel the longer
distances
necessary to collect fuel and also take care of other
household
tasks. Income-producing activities such as pottery-making
and
processing food for sale must be abandoned where fuel
becomes
too expensive. As land becomes cleared of vegetation, soils
degrade, ponds silt up, and valuable plants are lost. When
plants
are lost, traditional medicines based on this vegetation are
lost.
When the soil is less fertile, home gardens have lower
yields.
When ponds and lakes silt up, fish do not reproduce.
Without
nearby forage, small animals cannot be raised. All of these
factors affect the ability of an area to sustain life.
Factors affecting the adoption of energy technologies
The political as
well as the social, economic, and cultural
characteristics of a society affect whether new energy
technologies
are feasible and will be accepted. These characteristics are
often more influential in the adoption or rejection of an
energy
technology than technical soundness.
A National
Academy of Sciences study of the primary factors
affecting the acceptance of biomass-related technologies
found
the following conditions to be most important (NAS, 1982):
How well the
technology adjusts to the economic and financial
structures in a
society:
*
Who owns the resources the technology will
use?
*
What are the sources of capital for
financing a
technology
and who has access to them, either directly
or
indirectly?
*
What is the economic rate of return?
*
Is the economic rate of return greater for
this use
than for
alternative uses of this resource?
*
What are attitudes toward risk and how can
the risk
involved
in adopting a technology be minimized?
Even if a
technology is "given" to project participants as
part of the
project, a long-term commitment to use the
technology
depends on the availability of natural resources to
fuel the
technology, financing to maintain and repair it, and
the amount of
risk involved in changing. (See also French,
1979.) The
economic rate of return for the use as compared
with alternative
uses should be considered, although other
factors such as
social value may outweigh the economics.
How compatible
the technology is to the existing organization of
work:
*
What is the division of labor within the
family or
social unit
(by age, sex, ethnic group, etc.) affected?
*
How will the division of labor be affected
by a
proposed
technology?
These factors
affect whether the people who presently
perform the task
involved will be likely to find the
technology
beneficial in terms of its effect on time, the
patterns and pace
of work, and the social organization
related to this
work. A technology that disrupts the division
of labor among
men, women, and children is likely to be
resisted,
especially if it changes the access of sex or income
groups to
productive resources.
How well the
technology can be integrated with the existing social
structure and value
system:
*
What social customs, moral values, and
religious
beliefs
determine the way energy is used?
*
How can change accommodate these customs?
Traditional
lifestyles and ways of thinking can be
threatened by new
energy technologies. For example,
displacing the
traditional three-stone stove in the Sahel
requires
sensitivity to it as a symbol of harmony between a
husband and wife.
How well the
technology adapts to the local political system and the
decision-making
process:
*
How are decisions made and enforced in the
community?
*
How are disputes settled?
Obviously, the
answers to these questions will define a
hierarchy in the community that in itself may reinforce the
poverty of a participant group. Knowledge of the system may
for
this reason be all the more important, because open conflict
between a participant group and the local political
structure may
be too great a risk for the participant group. Minimizing
the
conflict will help mobilize the support of participants.
There are many
examples of the relevance to the political
structure of an energy project. A successful agroforestry
project
depends on the structure that assures the land-tenure system;
a
charcoal project depends on those who set prices and
regulate
distribution. Those that control the credit structure will
affect
the viability of almost all energy projects.
This is not an
exhaustive list of the various factors that
influence the adoption of an energy technology, nor are each
of
the factors listed necessarily of equal importance. You may
find
other factors in your community and some may be more
important
than others. (This discussion has not included the
environmental
factors that affect the adoption of energy technologies
since t his
is covered elsewhere in the booklet.)
Who pays for environmental problems?
Individuals or
groups that misuse resources may not be those
that experience the consequences. Some individuals may
realize
that they are engaged in practices that lead to degradation
of
resources that will directly or indirectly decrease land
productivity
over a period of time, but continue with the same practices
in order to survive from day to day. When degradation of
resources does not affect the people who cause this
degradation
or when poverty allows no other alternative, it is difficult
to
modify environmentally unsound practices.
In many areas,
energy resources are collected free of charge,
often on public lands. This includes crop residues, twigs,
wood
scraps, and dung.
When this collection adversely affects
resources, it is the community as a whole that bears the
cost, not
the individual. A charcoal-production example illustrates
this.
Charcoal-makers often use inefficient, inexpensive kilns,
and
migrate in search of wood. The incentive for them to invest
in
more efficient kilns is minimal because they do not bear the
costs
of the deforestation they may be causing.
Environmental
problems or benefits generated by one group
may be experienced by another group.
For example, siltation
results from the dispersal of topsoil and nutrients from
land (often
due to deforestation). The most direct and dramatic effect
is to
alter downstream watersheds.
Reforestation to remedy that
problem may be perceived locally as loss of land, but it can
result
in benefits to downstream farmers.
The
interrelationships within and between ecosystems are
responsible for the fact that those who cause environmental
degradation may be different from those affected. Remedies,
however, usually require a change in the practices of people
who
initiate the act that triggers a chain reaction in the
ecological
setting. It is important to develop acceptable incentives
for
change. When the poor are forced by socioeconomic
circumstances
both to cause and suffer from environmental degradation,
income-producing activities are needed to modify practices.
One example of a
project that is providing income-producing
activities is located in the Horn of Africa. Training,
seeds, and
materials are offered to individual refugees to encourage
them to
grow tree seedlings. The seedlings of a prescribed height
are
purchased from the trainees. Thus the project provides both
income potential and the opportunity to assist in reducing
the
environmental degradation to which the large numbers of
refugees
are inadvertently contributing.
Chapter IV
ENERGY
PLANNING FOR SUSTAINABLE DEVELOPMENT
Energy planning
for sustainable development is a process for
devising projects that use natural resources to meet local
energy
needs in a way that is socially and culturally acceptable,
environmentally
sound, and economically feasible. The purpose of such
planning is to avoid the pitfalls of energy projects that
are not accepted
by the people meant to benefit from them, that use
inappropriate
technologies, that ignore the environmental constraints
of the natural resource base to provide energy and other
resources
in the future, and that are not economically feasible.
Why plan?
The planning
process can serve a variety of purposes:
*
Identify potential community problems.
*
Help community develop solutions.
*
Uncover difficulties and benefits that might
arise through
a given
solution.
*
Set up a system for adjusting to unforeseen
effects that
may occur.
Good planning
creates a consensus among those affected by
the problem and the solution--the participant group. It is
essential that this group participate in the entire planning
process. This is particularly critical for small-scale
energy
projects since they are very localized and draw upon
resources
that local people use and know intimately, and that directly
affect their day-to-day survival. Because the value of
energy is
in the work it can perform, the tasks must be defined by
those
who will benefit from the work to be done.
Ineffective
planning may cause environmental problems by
not taking into account new pressures on resources that the
project itself can create. For example, dung used for fuel
may be
diverted from its use as fertilizer and deprive the soil of
nutrients.
Energy diverted from other uses for an income-generating
project such as food processing may require gathering more
energy resources than are locally available on a sustainable
basis.
Poor planning may also hurt the poorer groups, by reducing
their
access to various energy sources.
Special considerations in meeting energy needs
Energy needs are
for heat, light, and mechanical power.
Supplying these needs can be accomplished in several ways:
*
Managing and increasing the supply of energy
sources.
This can be
accomplished by tree planting on marginal
lands, managing
or creating village woodlots, or introducing
integrated
home gardening approaches to farmers.
Additional
energy based on the wind, the sun, and water
can be
developed. In situations where the supply of a
resource is
being depleted by non-energy use activities,
the planner
could attempt to introduce actions that
reduce this
loss. Deforestation losses as a result of
agricultural
expansion are an example. <see image>
esed8x37.gif (437x437)
*
Developing new conversion technologies:
Conversion
technologies
include solar and wind devices, small-scale
hydro
installations, and biogas digesters.
These
technologies
can open up new sources of energy or
increase the
efficiency of tapping existing sources.
*
Improving the efficiency of end-use
devices: The
efficiency of
devices that utilize energy can often be
improved
substantially. Cookstoves are a good example.
esed9x38.gif (393x437)
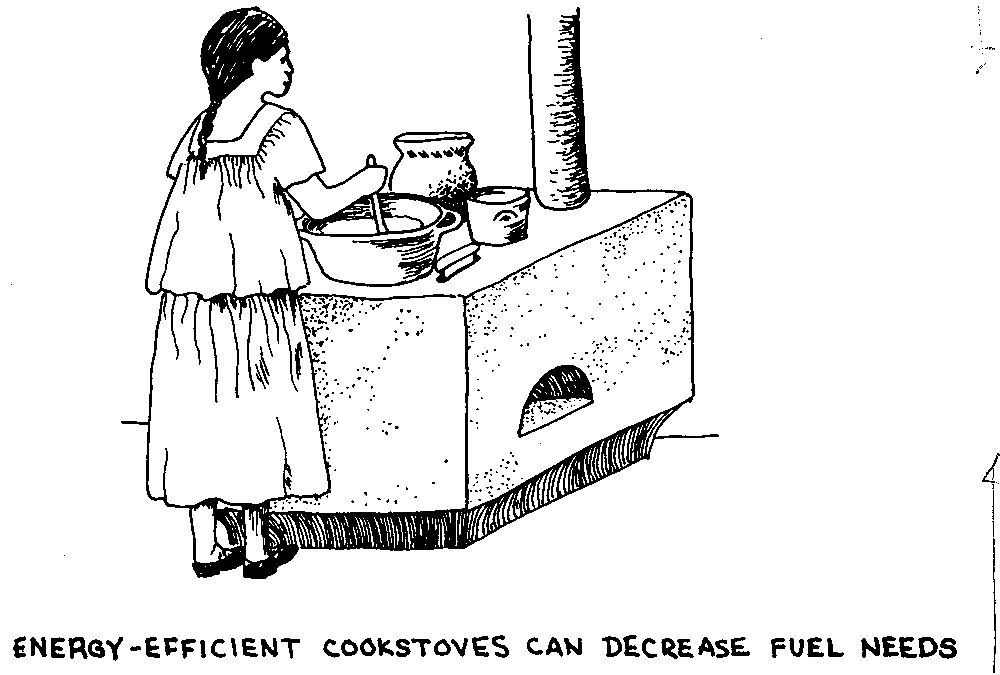
By developing
more efficient designs, less energy is
required. The
overall impact on energy consumption from
the
introduction of more efficient stoves and which
models are
more efficient are still being studied. This
will be
discussed more fully in a later chapter.
Simple
improvements in household and agricultural
implements
also fall into this category. While often
overlooked,
such improvements can greatly decrease the
amount of time
and human energy used.
*
Reducing energy losses and economic costs
that result
from
transporting and transmitting energy supplies. In
many cases the
energy is consumed in the process of
converting the
energy source to its end use.
The diagram on
the following page indicates the relationship
ese10390.gif (486x486)
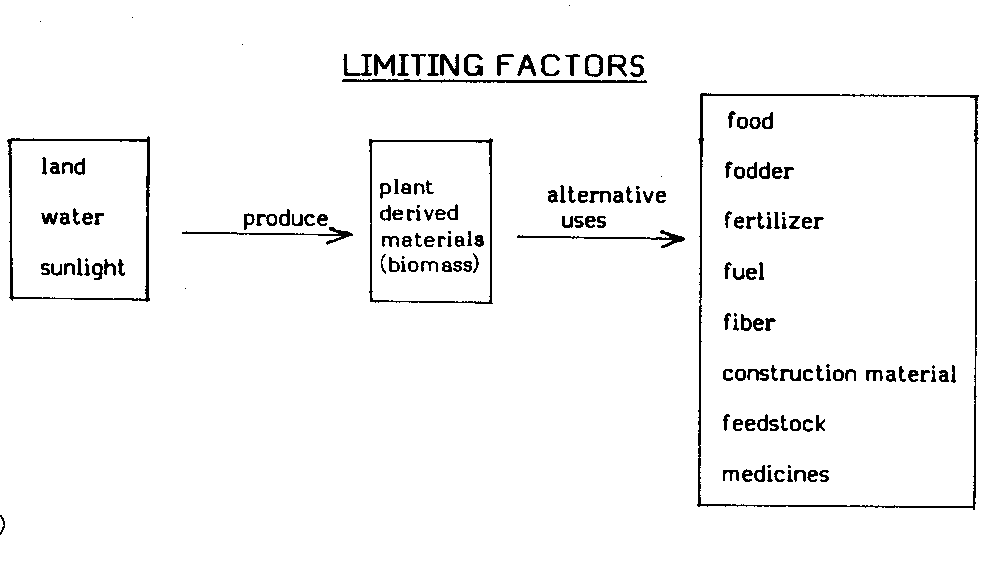
Which ways are chosen to meet energy needs depends on a
number
of factors. In the planning process, development workers and
communities can collaborate to assess present energy needs
and
supply resources available for development, and suitable
technologies.
Decisions will be influenced by what is socially and
economically
feasible, environmentally sound, and culturally acceptable.
In planning, the
different uses of potential energy sources
should be examined carefully. This will bring to light
whether
shortages will be created in using a resource for energy.
The following
diagram and set of questions summarize this process for
one important source of energy, plant derived materials,
commonly
called biomass.
*
What biomass resources are available in your
community?
*
How much biomass is used?
*
Flow is biomass allocated for these
different uses?
*
What are the competing uses for the
resource?
*
How will increasing its use as fuel affect
competing uses?
*
Will increasing its use as fuel mean that
the supply of
biomass will
be collected beyond its ability to regenerate,
and thus
create environmental problems?
*
What social or economic groups will be
affected by
changes in the
supply or price of energy?
*
Are there ways to overcome the environmental
and social
problems of
using more biomass for fuel by creating
additional
sources, developing appropriate conversion
technologies, and/or improving end-use
devices? Should
other
alternatives be considered?
Clearly, the
answers to many of these questions can only be
found by talking with people in the community, especially
the
women and the poor.
What is end use?
Energy is a means
to a specific end: to pump water, cook
meals, move material. Such tasks are called end uses.
Devices
that utilize energy are called end-use devices.
One energy source
may be able to provide for several end
uses. For example, wood can be used to cook a meal, to fire
a
brick kiln, or to provide light. Each activity involves
different
costs that determine whether the use of wood is economically
feasible. Each use
may have different impacts on the
environment, if the quantity of wood required or the manner
of
collection differs. The precise environmental impact will
vary
with the availability of wood and the condition of the
forest
ecosystem. It must be viewed within the broader context of
all
the activities which affect the natural resource base.
<see image>
ese11x42.gif (600x600)
One end use may
be powered by a number of energy sources.
For instance, transportation can be obtained from vehicles
powered by animals, petroleum-based liquid fuels,
electricity, or
even gasifiers. Each alternative energy source has different
costs, and its use has different impacts on the natural
resource
base. While similar work can be accomplished, vehicles--and
the
quality of transportation provided--will vary with the
different
energy sources. <see image>
ese12x43.gif (600x600)
How efficiently is energy used?
When one form of
energy is converted to another form there
is always some energy lost as heat.
The relative amount of
energy loss may be expressed as a "conversion
efficiency," in
which the smaller the loss, the greater the efficiency.
Technically, the
efficiency of energy conversion can be
measured by comparing the amount of useful work done by the
amount of energy required to do it.
Stated differently,
Energy
Efficiency =
Useful Energy Output
--------------------
Energy Input
Since output is never as great as input, this value will
always be
less than 1 (a fraction).
One of the main
reasons to measure energy efficiency is to
determine areas where research can be done to increase the
effective use of energy from a fuel source.
It also permits the
planner to measure the effectiveness of alternative
technologies
if and only if those measurements reflect actual use in the
home
or industry.
Large losses
always occur when heat is transformed into
mechanical energy.
This happens, for example, in the internal
combustion engine or when hot steam is used to turn an
electric
generator. On the
other hand, moving water to generate electricity
involves little heat so overall conversion losses are
relatively
small.
Matching energy
sources and technologies with their use (that
is, being energy efficient) is economically and
environmentally
sound. Mismatching
energy sources and uses is not only energy
inefficient, but if the resource is essential to the
ecosystems, the
use of an inappropriate source may have a negative effect.
Energy efficiency
is only one factor in the selection of
energy technologies.
The ease of transport, storage and use of
the resource; the availability and cost of the end-use
devices
needed to use the fuel; the amount of government subsidy;
cultural taboos; and considerations of cleanliness, smoke
content,
or other factors all help determine the final selection.
Unfortunately,
these other factors often overwhelm any
consideration of energy efficiency.
This has encouraged, for
example, the use of electricity for heating water, or the
use of
diesel fuel to power irrigation pumps when lower quality and
less
costly (both to the environment and the consumer) energy
sources,
such as solar water heaters or windmills, may have been more
appropriate.
In matching
energy sources with uses, there may still be
negative effects on the environment.
For example, while
methane from a biogas digester is a good source of cooking
fuel,
methane production has a by-product that is difficult to
dispose of
and that may cause environmental problems.
Measuring energy output
How does one
measure energy? How does one measure
different kinds of energy so that they can be compared? Can
the
energy stored in a tree be measured in the same way as the
energy available from a water mill? It is a very important
problem, because understanding the linkages between energy,
natural resources, and utilization requires that energy be
measured and evaluated correctly.
Several energy
sources can be compared by converting them
into common units such as Btu's and joules (See Appendix A
Energy Conversion Table).
For example, it is relatively easy to
compare gasoline with natural gas with vegetable oil.
Technologies like hydroelectric generators or photovoltaic
cells
can also be compared.
It is difficult,
however, to measure sources of energy that are
not readily converted into standard units of measurement,
such as
biomass or human and animal energy.
Clearly, there will also be
problems in comparing these sources with conventional
sources.
This poses a real problem at the community level where one
is
looking at all kinds of energy sources and uses in a
community and
trying to compare dissimilar things.
For example, some produce
heat, some produce mechanical energy, and some produce
electricity.
In some situations measurement can more appropriately be
made in terms of the time required to perform certain tasks.
What type of
energy data and what level of data should the
community planner collect? Following are some general points
to
help make such decisions:
1.
It is constructive to be as thorough as
possible. Find out
what data already exist.
Data from other surveys on
agriculture,
public health, forestry, or transport might
help to fill
in some of the gaps. There is no
universal
standard
regarding what constitutes sufficient data.
The
best standard
is to ask yourself continually whether these
data are
useful for the purpose at hand.
2.
Collect information that allows the planner
to:
-- identify
the supply or sources of energy
-- quantify
the interrelations between the energy
sources,
the people who use those energy supplies and
the
environment they live within
-- determine
how the energy is being used, the pattern of
energy
use, pathways of energy flow in the
community,
and factors that affect present and future
energy
use.
3.
Try to use measurements that allow
comparison between
energy
sources that might substitute for one another, or
between end
uses that employ similar energy sources.
For
instance, in
comparing the use of charcoal as a replacement
for fuelwood,
try to calculate both in terms of the
amount of
wood involved.
4.
Testing the efficiency of end-use devices
such as
cookstoves requires considerable
planning and attention to
detail.
An international committee of woodstove
technicians
has recently formulated a series of three
provisional
standard testing methods for wood-burning
cookstoves,
including water-boiling, controlled cooking,
and actual
field performance tests. Copies of the
test
procedures
are available from VITA (See Appendix E,
Sources of
Information).
In all cases,
testing should take place under the conditions
in which the
device will be used, as well as in the
laboratory. This should include
testing with the kind of
fuel to be
used, along with the people who will use it and
the uses for
which it will be required. Testing
under
laboratory
conditions alone may have little practical
relevance. For example, wood cut
and prepared in ways
people who
use a device are unlikely to follow may not
indicate the
same efficiency as a cookstove tested under
field
conditions.
The following
section outlines some of the problems in
measuring particular sources and offers some suggestions on
how
to avoid mistakes that are often made.
Wind:
Wind is extremely site-specific and varies
with the
terrain, season, and range of wind force.
Data should be collected
at the potential project sites, particularly for electricity
generating
applications.
General data for a region, (for example,
information collected at a local airport or weather station)
may
be helpful but should be supported by site-specific
information.
Solar:
Seasonal variation caused by cloud cover
should be
noted. Data should
be collected during the season when the
energy will be required for the end use.
It is not necessary to
gather data directly on the project site, as with wind.
General
data for the area are sufficient.
Water:
Three mistakes are commonly made in
gathering
information on water availability for hydropower:
*
gathering data only for part of the season
(even if the site
has reasonably
even rainfall, the river or stream's watershed
may not)
*
not calculating alternative demand for the
water adequately,
particularly
where, as with irrigation, it is an
intermittent
but critically important demand
*
not estimating sedimentation rates
accurately (sedimentation
quickly cuts
hydro capacity, and therefore blocks its
renewability).
Forests and
vegetation: Be careful not to equate
fuelwood
with logs. In rural
areas, most wood that is burned is scrap, twigs,
or dead wood.
Estimates of wood resources and cookstove
efficiencies based on tree trunks and large limbs have
resulted in
grossly inaccurate estimates of wood resources and the
potential
savings of wood from improved cookstoves.
Similarly, the
word "forest" should be used with care.
Fuelwood often comes not from forests but from the borders
of
farmers' fields, from grazing land, shrubs, and from pruned
high-value trees in fields around garden plots.
Estimating the
rate of fuelwood consumption can be
difficult. In urban
areas, where wood is purchased, it may be
sufficient to find out how much money various families spend
for
a bundle of wood, and how often they must purchase it.
It would
then be necessary to measure the average weight of such
bundles.
In regions where
fuelwood is gathered freely, consumption
estimates may be made by weighing the wood supply at the
beginning and end of each day.
Naturally, it is important always
to measure fuelwood use from families representative of the
regional population, allowing for market days, religious
observances, and any other events that can affect the daily
amount of wood consumed.
Other useful information includes
other uses for fuelwood besides cooking daily meals.
When the
results are expressed in terms of weight of wood consumed,
it is
important also to note the species composition, relative age
of
the tree, whether the tree was cut live or fallen, and
average
moisture content.
Crop
residues: Estimates of crop residues
should include a
careful calculation of seasonal variation.
In some areas of West
Africa, for instance, fuelwood surveys were inaccurate
because
the method of data collection ignored the four to five
months of
the year when rice straw and other crop residues were
substituted
for wood. If
possible, the net flow of crop residues should be
estimated, and some determination made of the present use of
these materials in recycling nutrients back to the soil.
Animal
residues: Dung supply can be crudely
estimated by
calculating the number of animals owned, or available, near
the
project site.
However, there may be considerable differences
depending on the health of the animals, the feed, and other
variables. Some
observation and data collection are essential,
especially on the location of the dung (is it distributed
over a wide
area, are the animals kept in a closed pen, are they brought
in at
night?)
For information
regarding specific questions about data
collection write to VITA (See Appendix E, Sources of
Information.)
Chapter V
ULIPUR, BANGLADESH: A CASE STUDY(*)
This study shows
how energy uses and sources by different
income groups in a village setting are interrelated.
The way the
study was put together may prove useful to planners in
thinking
through how to present an accurate picture of energy
production
and use in a dynamic situation.
This is an important part of the
energy planning process, because it provides the basis for
seeing
how an energy project may affect the local ecosystem and
different income groups.
Of the 2,300
people in Ulipur, 330 were selected for a
detailed review of their energy use.
Two kinds of information
were collected:
*
Socioeconomic data:
The family structure, resource
ownership
patterns, and productivity of the land and
animals.
*
Energy use data:
What energy is available, and how it is
used by
different income groups.
How were socioeconomic data collected?
Field workers
talked to families to learn the relationships in
the household, the names and ages of family members, their
sources of employment, and how much they earned.
The field workers
asked about the families' animals: how
many they owned and the size of their animals.
The researchers
(*) See Briscoe, 1979 and deLucia, 1982.
measured the amount of land owned by each family, and found
out
how much was farmed by the family itself, how much was
farmed
by non-family members, and how this was arranged.
They also
asked how a family was compensated for letting their land be
used
by someone else. <see image>
ese15x51.gif (486x486)
The field workers
talked to families about what crops were
produced on their land, crop yields, and whether the crops
grown
were used for themselves, sold, and/or given to other
families.
They asked families the quantity of crop residues and how
they
were used.
*
Did the family collect them?
*
Did they allow others to collect them?
*
Did they use them or give them away and to
whom?
This information
was then analyzed. The villagers were
classified as to whether they were landless, poor,
middle-income,
or rich. The field
workers found that the rich, comprising only 16
percent of the families, owned 83 percent of the trees, 58
percent
of the crop land, and 47 percent of the cattle (see the
table
below). They also
found that while families of different income
groups used the same quantity of fuel per person for cooking
food,
different types of fuels were used by different income
groups.
OWNERSHIP OF FUEL PRODUCING RESOURCES
Families Land
Tree
Cattle
Number %
%
% %
Landless
22 45
2
5 5
Poor
11 23
13
5 24
Middle-Income
8 16
27
7 24
Rich
8
16 58
83
47
Total
49
100.0 100.0
100.0
100.0
How were data collected on energy use?
Every two weeks,
the field workers went to see the families
to get information about the previous day.
They weighed the
cattle dung and talked with the farmer about the use of the
animal on the day before and how much dung was produced
during
that activity. They
asked how the dung was used or would be
used. They also
discussed the type, source, and amount of cattle
fodder used; how much milk the cattle produced; and how much
time was given to care of the cattle.
At the same time,
the field workers tried to estimate the
amount of human and animal energy expended during a day.
They
talked with the family about the previous day's work. What
kind
of work was done and how long did it take? How many people
were involved and how many animals? Was the work done for
their own fields, or did they also spend time working in
some
other family's fields? Did they have non-family members
working
in their fields?
When they worked
for another family, or another family
worked for them, how were the people paid? Did they get
cash,
food, or fuel, and how much?
It was also
important to determine crop yield. Families were
asked about what had been planted over the last two weeks.
They
discussed the use of fertilizers:
* What fertilizers
were used?
* Where were the
fertilizers obtained?
* How much was used
and for which crops?
Together, the farmers and field workers estimated how many
crops were harvested, and talked about how the harvested
crops
were used. Were they eaten by the family itself, were some
sold,
were some given to non-family members for work or some other
service? The farmers and field workers discussed crop
residue use
as well:
* How much did the
family use?
* How much was
gathered for use by non-family members?
* How much was left
in the field or just burned?
Each few months,
the field workers spent the whole day with
a family to observe the use of fuel in cooking all the meals
of the
day. They weighed the fuel used for each meal, estimated the
amount of food cooked, and counted the people fed.
Other sources of
energy and their use were also discussed and
noted. From these pieces of information a picture of the
energy
sources available and their use began to emerge, and a table
of
annual fuel use in Ulipur could be constructed.
ANNUAL FUEL USE IN ULIPUR
Percent
Crop Residues (from ten crops)
59.2
Animal Residues
2.7
Firewood (including twigs and branches)
From village
trees 10.8
From the river
4.4
Purchased
5.2
Subtotal
20.4
Other Fuels
Doinshah
(legume)
4.9
Bamboo
3.6
Water
Hyacinth
1.6
Other crop
residues and leaves 7.6
Subtotal
17.7
TOTAL
100.0
The information
collected showed that the rich had access to
almost twice the amount of energy (straw after livestock
feed,
jute, dung, and firewood, leaves and twigs) that they needed
for
cooking. The landless had access to only about 15 percent of
their
fuel needs for cooking. The rest of the energy they needed
came
from foraging for firewood, leaves, and twigs on public
lands or on
land owned by others. The landless were dependent on the
rich
for fuel and food in exchange for labor.
The landed poor,
who required all the dung produced by their
cattle (and more) for fertilizer, relied for cooking mostly
on rice
straw left over after the livestock were fed. Since the rice
straw
did not meet their needs for cooking fuel by about 13 percent,
the
poor were forced to bridge this deficit by spending valuable
time
foraging for twigs, leaves, and firewood and selling their
labor
during peak agricultural periods to the rich in exchange for
fuel
and food. They, along with the landless, were dependent on
the
rich. Of ten, their own crops suffered.
By looking
carefully at the information on the sources of
energy available in Ulipur and the uses they are put to for
both
energy and other purposes, the field workers constructed an
energy flow diagram of Ulipur. This diagram masks the
seasonal
variation in energy availability, but shows the complex
interrelations among resources as well as many uses each
provides.
How to use the energy flow diagram
The energy flow
diagram brings out the multiple and competing
uses for particular resources. One is thus able to predict
the effect on the ecological, social, and economic system of
the
village of using more of a particular resource for energy.
Suppose that the
villagers expressed a need for night-time
lighting and a more efficient cooking system. Suppose also
that a
biogas system based on animal waste is proposed. The energy
flow diagram, along with the data on energy use by income,
can
help in thinking through the implications of biogas for the
resourse system prior to project design and implementation.
Let's think this through together. <see images>
ese16x56.gif (486x486)
In Ulipur, 62
percent of dung is used for fertilizer, 13 percent
for fuel, and 25 percent is uncollected and probably
uncollectable. The dung used as fertilizer has probably
shown
itself over time to be the amount needed to condition and
enrich
the soil; using any of this 62 percent of the dung would be
likely
to jeopardize agricultural yields. The rich have the use of
almost
all of the dung used as fuel.
The distribution of
resources implies that the rich would
benefit most from a village biogas digester. They are the
primary
group using dung for fuel and so they already have the
necessary
raw material. Middle-income and poor families would need to
divert some of the dung presently being used for fertilizer
to the
biogas digester. This could diminish crop yield for these
two
groups. Also, the use of dung for the biogas digester would
increase the value for what was previously a free or
low-cost
good. This would hurt the poor who depend on its being
freely
accessible.
The ecological
effects could be serious. If the poorer people
decide to use dung for fuel rather than fertilizer, the
fertility of
their land could be reduced. A decline in rice production
would
reduce other fuel sources since rice straw provides about 75
percent
of cooking fuel and jute stick provides about 15 percent of
it. Less rice straw and jute stick would intensify
competition
between using them for energy and for other purposes, since
jute
stick is also used for construction, and rice straw to feed
livestock.
If livestock are fed less, they produce less dung for use as
fuel or fertilizer, further decreasing crop productivity.
The
landless and very small land holders might be forced to get
more
firewood, which generates another set of environmental
problems.
To address some of
these problems at the beginning of a
biogas project, one should ask:
*
Would the poorer parts of the community get
enough
energy from the
biogas digester so that they would not
use for energy
dung that is required as fertilizer, or use
crop wastes that
can have uses other than energy?
*
Would the poor have access to the sludge
by-product
produced by the
digester that can be used for fertilizer?
*
Could the digester, by supporting an
income-generating
activity,
provide enough income so that there would be
other ways to
obtain energy and fertilizer?
Summing up
The work described
above in Ulipur was undertaken over the
course of a year. Any village energy project must be
preceded by
study or collected knowledge of annual cycles and seasonal
variations that can influence the demand for energy and
availability of resources. The process of talking with community
members in order to gather relevant data about socioeconomic
relationships and resource distribution and use is a
necessary one.
Making use of existing data and consulting with others can
often
shorten the process but it should never be solely relied on
for
information.
Chapter VI
A
PROCESS FOR PLANNING ENERGY PROJECTS
Ideally, a planning
process follows a logical sequence of
activities, each of which builds on another. It begins with
information gathering and discussions with the participating
community. As community workers and the community interact,
needs, general goals, constraints, and options emerge.
Projects
develop as community workers and the community think through
needs and goals, and how to attain them.
It is essential
that community workers and local people
devise a variety of approaches that will suit their goals
and deal
effectively with any anticipated constraints. From these
alternatives the most suitable one can be selected as the
project.
During
implementation and operation, the project can be
monitored to ensure it continues to meet its goals and to
enable
the community to resolve any problems that may arise.
Finally,
once the project is complete, it should be evaluated to
determine
if it was successful and to aid in the planning of future
projects.
The diagram on page
64 shows the steps involved in the
ese18x64.gif (540x540)
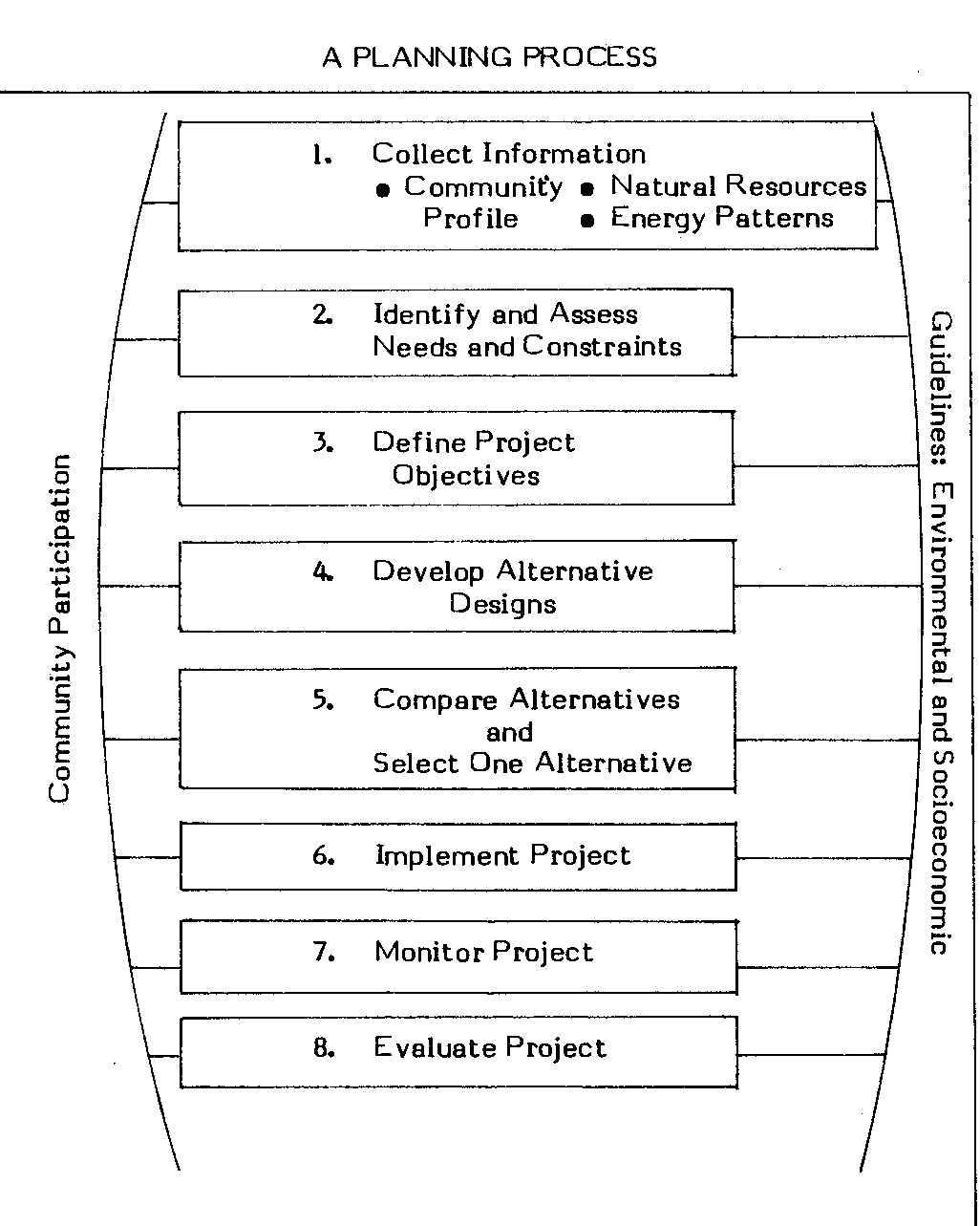
planning process. Each part of the process will be examined
in
detail in this chapter, especially as it applies to energy
projects.
Community participation and environmental and socioeconomic
guidelines are integral parts of each step in the process
and will
be considered first.
Community participation
To establish a
successful energy project, the community must
participate fully in all aspects of the project. The project
must
address the needs of the community. As a source of
invaluable
information about the environment and local practices, the
members of the community must be consulted. If the project
is
endorsed by the community it is more likely to meet the
needs
and to be adopted.
Communities,
however,
ese17x60.gif (353x285)
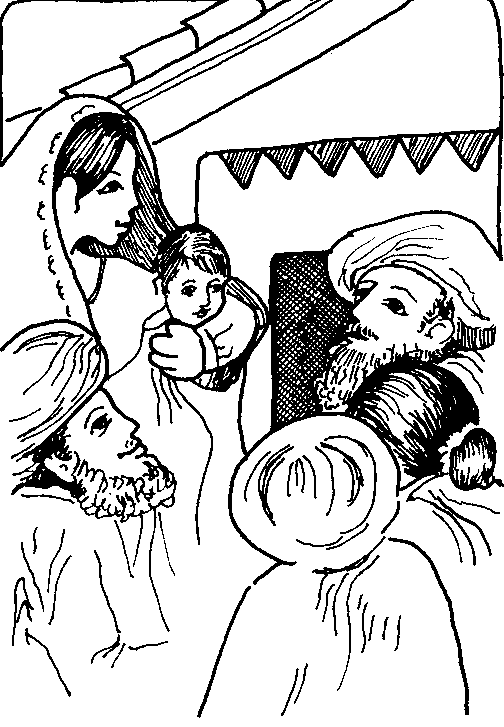
are groups of individuals,
some of whom may have
conflicting goals. Projects
that address the goals of
those with similar or at
least non-conflicting goals,
should also take into
account the interests of
non-participants in order
to achieve equity.
During the initial
discussions with the community,
local issues of greatest concern will become apparent.
Energy may emerge as a priority, it may be only indirectly
related
to the central problem, or it may not be an issue at all.
Often
projects fail because they are not directed to local
priorities.
For example, a
project to prevent desertification and provide
fuelwood in Senegal was devised by foresters without talking
with
the villagers. The villagers were asked to plant trees
around
their gardens. When no one planted trees, forestry officials
thought the villagers were lazy and ignorant. In later
discussions,
it was discovered that the villagers thought the gardens
were not
worth additional time because there was not a way to get the
produce to market. To generate interest in improving the
gardens, the need for roads and marketing infrastructure
should
also have been considered. One should establish that energy
is a
local priority before proceeding to plan an energy project.
Environmental and socioeconomic guidelines
Guidelines suggest
those things that should be considered in
designing, implementing, monitoring, and evaluating a
project.
Guidelines raise questions that will assist the planner to
avoid
pitfalls and to maximize possibilities. Guidelines are
different
from goals. For example, a goal might be to provide energy
for
lighting a school; a guideline would be to make use of local
resources in providing energy for lighting.
Social, economic,
and environmental factors may need to be
weighed against each other to balance the advantages and
disadvantages
in these areas. A useful tool for examining relevant
factors in relation to the project is Fred Weber's
Ecological Mini
Guidelines, which is included as Appendix B.
For example,
economics may determine an energy project's
feasibility, and the environmental benefits it will produce
may
make it attractive to development workers. But if the
project
does not grow out of community-voiced decisions, or if it
cannot
be operated, maintained, and monitored by the community, the
social guidelines may dictate that the project should not be
undertaken.
Below is a short
list of some of the environmental and
socioeconomic guidelines for energy planners. The list is
not
exhaustive but offers a general framework of the types of
guidelines that may need to be considered in designing a
project
that best serves the needs of the community involved.
Environmental Guidelines - Environmental guidelines evaluate
the
community energy needs as they relate to the natural
resource
system.
*
Identify the competing uses for the
community's natural
resources.
Determine the appropriateness of using each
resource, while
considering the effects of its use.
*
Use an integrated planning approach that
places a high
value on
natural resource management. This will allow
the planner the
opportunity to develop energy projects
that manage
resources rather than simply consume them.
*
Consider how the project will maintain or
enhance the
ecological
productivity of the natural resource base used
to produce
energy.
*
Consider the need to use natural resources
on a long-term,
sustainable
basis.
*
Think of energy in terms of the purposes for
which it will
be used.
Integrate energy planning with agricultural
projects when
appropriate because the natural resource
system must
provide both food and energy.
*
Develop energy projects that reduce erosion,
maintain soil
fertility, and
protect watersheds.
*
Develop energy projects that take into
account the
seasonal
availability of and demand for water, crop
residues, and
wood so that use does not exceed supply.
*
Maintain or enhance water supply and quality
by, for
example,
maintaining watersheds or taking care in the
disposal of
waste materials.
*
Build into the project the length of time
necessary to
replenish the resource used for energy,
being careful to
consider the
demands other than energy that are being
placed on the
resource.
*
Identify the ecological values in
traditional practices and
apply them
where possible.
Socioeconomic Guidelines - Socioeconomic guidelines help to
incorporate the energy project into the local cultural and
institutional structure to help ensure proper operation and
maintenance.
*
Involve all people who will be affected in
all stages of
energy project development.
*
Make sure that the use of a natural resource
for energy
does not affect
its use by the landless and very poor, who
will be worse
off and forced to over use other resources
to meet their
energy needs.
*
Build upon the existing social organization
and customs
for
environmental rehabilitation and conservation.
*
Develop land use strategies that minimize
conflicts
between energy
and agricultural goals. Integrating energy
projects and
food production projects will help.
*
Develop energy technologies that provide
multiple uses
(such as a
biogas system for energy, fertilizer, and waste
management), so
that maximum use is made of the
investment and
the resources.
*
Develop energy sources that are most suited
to the task
both in terms
of cost and energy quality so that resources
are used
efficiently.
*
Balance health problems with other benefits
in designing
energy
conversion devices; for example, smoke from
cookstoves may
create respiratory problems but it may
also kill
problem insects.
*
Design projects that guarantee that the
target population
will have
control of the energy source or energy end use.
As noted earlier
these guidelines are not exhaustive. You
may think of others to add to the list appropriate for
project
planning in your area.
Steps in the planning process
1 Collect
information
The profile of
the community consists of the socioeconomic
organization, the way it produces and consumes energy, and
the
status of its natural resources.
This information can be a very
helpful planning aid.
It should be designed to provide easy-to-use
data on key social, cultural, ecological, and economic
characteristics.
The data should be carefully selected and the rationale
for gathering them should be made explicit.
Local people are
extremely important in helping to identify relevant energy
relationships as well as in helping to gather and analyze
information.
Early discussion with community members will serve to
direct the planner to certain problems, but a good planner
will not
form any conclusions regarding needs at this point.
There are two
purposes to this step in the planning process.
One is to determine the existing conditions.
The second is to
collect information that will permit the planner to quantify
the
relationships among energy use, natural resources, and the
people
who use the resources.
Often, local
people prove to be an invaluable source for such
information. In other cases, however, it may be necessary to
consult technical documents to obtain data on
characteristics
such as to the amount of insolation (soler radiation) in an
area or
imported energy use.
When properly collected, this information
can help save extra project costs.
The data should
be organized to provide easy-to-use
information on key social, cultural, ecological, and energy
characteristics.
Several types of information that should be
collected are outlined below.
*
Community profile--socioeconomic
characteristics
-- Who are the
people using the resource?
Examples:
Population size, growth rate, diversity, and age
groups
Number
of households
-- Who or what
affects/controls access to the resource?
Examples:
Land
ownership and land tenure system
Indicators of average income per household
(roofing materials, painted or white -- washed
building, number of animals)
Employment information, specifically in-house
and
rural industry sources
Available credit mechanisms for energy projects
(credit
mechanisms may only be available for
agriculture, check to see if these can be applied
to
energy)
-- What is the
local management system (actual and potential)?
Examples:
Community structure including leaders, economic
status,
etc.
Cultural traditions, attitudes, and perceptions
related
to energy sources and natural resources,
and
their uses
-- What are
the outside forces affecting local resource management?
Examples:
National, regional, and local policies that affect
energy
use and supply (laws, taxes, subsidies)
Regional and national energy markets, population
centers
-- What
factors affect the energy supply?
Example:
Agricultural practices
-- What are
the public health considerations?
*
Natural resources--ecological
characteristics
-- What are
existing uses of natural resources?
Example:
Land
use patterns, particularly agricultural land
and
forested areas
-- What is the
physical environment?
Examples:
Soil:
composition, organic content, ground cover,
erosion, use of local fertilizers, and steepness of
slope
Water: local sources, quality,
amount and
seasonal variability of rainfall and stream flow,
condition of watersheds, ground water supplies,
and use
Climate: annual temperatures, seasonal floods
and
droughts, amount and seasonal variability of
solar
insolation (energy that reaches the earth),
maximum and minimum wind velocities,
and
seasonal variations
-- What is the
biological environment?
Examples:
Flora
and fauna: vegetation (stable, changing,
balanced, requirements, and limiting factors for
regeneration), feed and water requirements of
animals
Biological communities in the area: composition,
diversity, stability
Biomass: amount of natural standing forests and
forest
residues; amount of trees and shrubs outside
of
forests, in open rangelands, around agricultural
fields,
in home gardens, along roads;
types
of crops grown; crop residues and seasonal
availability
-- How are the
natural resources being used or managed?
*
Energy Use Patterns
-- What are the
energy characteristics of this community?
Examples:
Energy
sources: present and future energy
sources
in terms of quantity, price, location, and
variability of supply of biomass, biogas, hydro,
organic
wastes, agricultural residue
Energy
conversion/process pathways: i.e., what
happens
to the energy between the source and the
final
end use, how is it transported, transmitted,
or
converted, etc.
Energy
end-use patterns: how is energy being
used,
how much is used for cooking, heating,
lighting, rural industrial use, household use, etc.
Organize this information by both cost and the
social
classifications (household/industry use,
income,
geographic location) identified above
Imported energy: amount, prices, and variability
in
supply of electricity, liquid fuels (e.g., gasoline,
kerosene, diesel), gaseous fuels (e.g., propane),
and
coal. Measure the time required for
energy
collection; Identify the producers and middlemen
for
energy and their role in the community
It may not be
essential to collect all of these data.
The
specific data that are important to the development of an
energy
project will often be determined as the development worker
and
the community jointly assess community needs.
2 Identify energy
needs and constraints
After examining
the information identified and collected for
the profile of energy patterns, some further refinements may
be
needed before determining the needs of this community and
the
constraints on those needs.
The following
should be explored about each energy source:
*
how much energy is used directly and how
much is
converted for
use in households, agriculture, small-scale
industry, and
transportation (including where the resource
comes from and
whether there are seasonal variations in
type and
quantity)
* trends in
energy consumption patterns, costs/benefits
pricing, energy
intensity for particular end-use functions,
and
energy-economy relationships
* energy
efficiency in key end-use devices
* competing
non-energy uses of the natural resources used
for energy: how
much is used for food, fodder, fertilizer,
fiber, or
construction; by whom
* changes in the
demand for, availability of, or access to
resources.
The assessment
should also provide information on what
groups of people are using the various energy types, how
they are
using it, where the sources are, what the seasonal patterns
of
supply and use are, and how much it is costing.
It is essential
to determine the factors that are or will affect
future availability of sources.
For example, predictions of future
energy needs may be based on observations of declining
supply or
increasing costs.
Analysis of the
relationships between the energy source,
competing uses of that resource, and the overall stability
of
natural resources is often ignored.
An adequate analysis of
fuelwood supplies might indicate that the effects of using
land for
agriculture would deplete fuelwood sources.
And the situation
would grow worse as population increased.
Analysis would allow
energy planners to focus on the causes of the problem rather
than
designing solutions that address the results of trends.
It is essential
to remember that current energy use occurs in
the midst of several interrelated and dynamic socioeconomic
and
environmental processes.
Too often solutions for energy problems
are based on technological perceptions.
This can be avoided by
planning projects that match the management of resources
with
the demand for energy that promotes development.
The planner
and the community must look at energy needs in this broader
context.
The information
will help the community to identify specific
energy problems that can be remedied through small-scale
projects. During the
identification process, the community may
find that potential energy sources are not being used for
energy or
that certain resources are being over used, which in turn is
resulting in environmental problems.
The socioeconomic
analysis will help the development worker
identify the groups that exist in the community, which of
them
control access to resources, what outside factors affect
access
to those resources, and the costs of those resources.
This will
allow the planner and the community to compare the energy
needs
of different socioeconomic groups and to predict which group
of
people will likely benefit from a proposed project.
An important part
of assessing needs is identifying
constraints -- the technical, economic, social, and
environmental
factors that restrict efforts to meet local energy needs.
This will
allow the planner to identify the factors that will impede
or
promote future development efforts in general.
For example, if
energy is not available or is inadequate for water lifting
for
irrigation, this might be considered a technical constraint.
In Indonesia,
subsidies on kerosene acted as an economic constraint
to fuelwood management.
As a result of the low price of
kerosene, demand for biomass declined, which in turn
contributed
to a lack of management of fuelwood supplies.
When the subsidy
was removed, an increase in the demand for biomass led to
increased prices for those resources.
Because other fuelwood
supplies were not available, the consumption of crop
residues
increased dramatically.
People did not begin to plant fuelwood
species to meet the growing demand until the price of
fuelwood
increased. Efforts
to increase the fuelwood supply while kerosene
was being subsidized would not have succeeded because the
government pricing policy was acting as an economic
constraint.
An example of a
social constraint can be found in Sri Lanka.
For religious and cultural reasons, dung is not considered
acceptable
for use as fuel. And
in other countries, researchers have
found that a lack of access to or control of a resource may
be a
constraint to efforts to provide energy supplies by
encouraging
tree planting. Where villagers do not own the land they farm
or
the trees that are on their farms, they have little or no
incentive
to manage what they may not be able to use.
Environmental
factors can also act as a constraint to energy
supplies. For
example, cultivation of marginal lands often uses
the same farming practices that were used on productive
soils.
And often, these farming practices are inappropriate for the
site.
The clearing of land results in a reduction of potential
biomass
energy supplies, increased rates of soil erosion as a result
of the
lack of ground cover, and a depletion of nutrients in the
soil. This
reduces the amount of water that can be stored in the soil
and
increased flooding frequently occurs.
The subsequent degradation
of the watershed then seriously threatens the water supplies
in
the area, constraining the successful introduction of a
hydro
project.
Additional
examples of constraints include a poor match of
an energy supply with an end use.
This may occur when rural
electrification is proposed for an area where the major
energy
need is for cooking.
Inadequate supplies of water or wind for
hydro or wind energy projects are examples of technical
constraints. The
cost of technologies, pricing policies, and
subsidies can all act as economic constraints to energy
supply.
Planners must be aware of the wide range of factors that may
constrain the supply, use, development, and management of
energy resources before they can successfully propose
solutions to
alleviate local problems.
3 Define project
objectives
The next step is
to formulate objectives for a project that
will be undertaken to meet the needs given the highest
priority.
Project objectives should serve the needs of the community
for
improving the quality of life.
Technological solutions should be
secondary in determining objectives. Combining energy
development
with natural resource management can contribute to
effective local and regional development.
Supplying the energy
needs of a community can have several components and a
single
project may be only one of those components.
Objectives may be
defined that help solve several problems in a region.
For example,
a project that provides electrical energy to a community may
also provide employment, and thus, a guaranteed market for
energy
from biomass. This
energy could be supplied from fuelwood,
agricultural wastes such as sugar cane by products,
industrial
wastes from milling operations such as wood chips and fiber,
etc.
Such a project could promote management of biomass sources
that were previously neglected by providing a needed
economic
incentive.
Project objectives
must be clearly defined: for instance, if
the goal is to increase energy supply, one specific
objective may
be to provide seedlings of fast-growing tree species to 123
families. This
objective may be further defined by indicating a
plan for training 10 farmers to grow these seedlings.
Thus, a
clearly-defined objective not only sets the task precisely
but also
provides a standard by which the project can later be
eveluated.
The guidelines at
the beginning of this chapter can help
determine the requirements for meeting the project's
objectives.
For example, if a guideline for developing energy
technologies
that provide multiple uses is adopted, the project might
include
growing trees that can be used for livestock feed and
construction
materials in addition to supplying energy.
Such a project might
also have associated environmental benefits by providing
erosion
control on steep hillsides.
In another
example, community members may voice strong
concern over the need for both erosion control and more
fuelwood
while the development worker's assessment of the resource
and
climatic conditions may indicate a need for watershed
management.
The community and development worker must then decide
which need has a higher priority, given the range of
technical,
social, and economic conditions present.
4 Develop alternative
designs
Once objectives
are defined, alternative designs for implementing
the project can be considered.
One of the first steps in
developing designs is to examine each identified need in
terms of
the effort required and the kinds of resources necessary to
meet
it. In many cases,
the development worker may want to seek
some additional assistance if the problems indicate a need
for
special knowledge.
If one of the alternative designs includes a
small-scale water installation, for example, consultation
with
hydropower specialists, water resource managers, and health
specialists may be necessary.
In general, a variety of opinions is
always helpful in reviewing decisions in order to identify
and deal
with possible problems.
The design of the
alternatives should be based on the
community's identified needs.
It should be consistent with the
environmental, social, technical, and economic guidelines,
as well
as technically feasible or appropriate.
Consideration of the
constraints will help to identify conditions that restrict
the
present energy situation or may limit the effectiveness of
the
project.
5 Compare
alternatives and select one alternative
Evaluations of
possible projects can be made at various
stages in the planning process.
In the early phases of designing a
project, an inventory of local and non-energy technologies
that
meet identified needs can be matched against the technical
resources available at the project site.
Many inappropriate
technical solutions can be eliminated at this planning stage
based
on the constraints already identified.
These might include an
inadequate supply of a resource (wind, water), excessive
costs,
lack of technical skills, etc.
For those solutions that are feasible,
an analysis of the benefits and costs of a project should be
made.
The analysis is based on a comparison of the alternative
designs
and uses criteria
derived from the previously-mentioned
guidelines. These
can be summarized as:
*
Economic and financial analysis: a thorough
evaluation of
the costs and
benefits of a project from the viewpoint of
the community
and its individuals should be conducted.
This should
address the long term concerns of a project's
ability to be
sustained: will the project succeed in the
absence of
economic support from outside the community?
*
Analysis of technical feasibility: a
thorough evaluation of
the technical
application of a given technology must be
conducted at
this stage. The most critical question
to be
asked at this
stage is whether or not the alternative
energy
solutions are appropriate to meet project
objectives. Additional questions
include whether the
technology is
proven or if it is still experimental, can it
be adapted to
the local conditions, are the raw materials
available, can
parts be located if needed, etc.
*
Assessment of social and cultural impacts:
technologies
that require
substantial changes in the social, legal, and
cultural
institutions in a project area will often be found
unacceptable
and end in failure. However, the
planner
should not
assume that a new technology will not be
readily
adapted because of social and cultural reasons.
SAMPLE BENEFITS/COSTS
DATE___________
ANALYSIS CRITERIA PROJECT
DESCRIPTION________
ECONOMIC
RETURNS
Self-Sufficiency. Rank high a
project which can be shown to lead
to jobs, skills,
training, improved markets or other economic gains which
are returned
directly to the community and can be shown to increase local
self-sufficiency.
Move toward the lower end of the scale if a project must
rely on continued
subsidy and/or it becomes less clear that the economic
gains will be
returned to the community.
Funding
Availability. Rank high a project where
funds are available
quickly and
easily (perhaps from local sources). Move toward the middle for
projects where
some funding is available but additional funds must be
sought. Use the
lower end of the scale in cases where funding is not
readily available
and a long time lag seems likely.
Net Profit.
Rank high a project where careful
calculation of
economic factors
indicates that the product or project will bring in more
than it cost.
Move lower on the scale as the project's economic
profitability
appear less clear.
TECHNICAL
RESOURCES
Local Techical
Support. If the project requires involvement of
change agents,
technical support groups, extension services, and these are
available, rank
high. Move toward the opposite end of the scale as
availability and
access to such support becomes less clear and/or difficult.
Technology
Availability. Rank as high a situation
where the
technology exists
and seems adaptable to the situation. Move toward the
lower (costs) end
as the technology requires more extensive commitments to
research and
development. Rank high situations where technology makes
maximum use of
local human and material resources. Move lower toward the
opposite end as
resources must be obtained from outside sources and this
could cause
delays and/or failure to use local resources adequately.
Technical Impact.
Rank high a project in which the technology or
project
once launched can be maintained by local
residents--this implies
training in
upkeep and repair and arrangements for replication. Move lower
on the scale in
situations where provision for these activities has not
been made. Rank
high a project which introduces a technology which seems
to require little
change in everyday life. Move toward the
lower end as
the technology
seems to require alterations in lifestyles, culture,
traditional
patterns, etc.
SOCIAL AND
CULTURAL ENVIRONMENT
Community-expressed Need. Rank high a project based on
community-expressed need. Move toward the opposite end as community
involvement in
need identification becomes less clear.
Social
Returns. Rank high projects which can
be shown to bring
cultural and
social gains to the community. Move toward lower end as social
and cultural
gains become less clear and/or the effects of the effort seem
likely to be
socially or culturally descriptive.
Rank high a project which
enables residents
to participate with least risk. Move toward the lower end
of the scale as
it becomes clear that participants run more risk, i.e., as
their investment
demands a level of commitment which would have serious
consequences were
the project to fail. Assume for project feasibility that
the smaller the
degree of change required in local custom, the easier it
will be to get
the project underway. Rank as high projects which require
little change;
move lower as more change is required.
NATURAL
ENVIRONMENT
Relevance to
Guidelines. Rank as high a project which meets all or
most of the
guidelines for an ecologically sustainable
activity. Move
lower as the project fails to meet these guidelines.
Use of
Alternative Control Methods. Rank high a project which makes
maximum use of
biologically sound control measures; move lower as the
project must rely
on chemical control methods.
Alternative
Design #1 (Costs) 1
2
3 4
5 6
7
8 9
10 (Benefits)
-
+
economic
returns
___________________________________________
technical
resources
___________________________________________
social/cultural
___________________________________________
physical
environment ___________________________________________
Alternative
Design #2
economic
returns
___________________________________________
technical
resources
___________________________________________
social/cultural
___________________________________________
physical
environment
___________________________________________
*
Assessment of environmental impacts: the
proposed
alternatives
should be evaluated to determine if they will
have any direct
negative impacts on the environment.
Will the
projects have negative secondary effects? Often
indirect
effects can be far greater than primary ones.
Extensive
checklists exist that the planner should use to
determine
actual impacts. Few projects properly
estimate the
economic costs of environmental damage
and this should
be done at this stage. Projects should
also
contain a plan
to mitigate such damages. Properly
planned projects
may result in improved management of
natural
resources, which will have significant long-term
benefits to the
community.
Each of these
criteria should be considered in relation to
each of the project designs.
In addition, there are some general
points that should be considered:
*
What are both the long-term and the
short-term effects
of the project?
*
Will meeting one criterion mean that another
cannot be
met, thus
making the project infeasible (e.g., will making
the project
economically viable have negative effects on
the
environment).
*
Is another viable alternative for meeting
the community's
needs lacking?
*
What would be the effects if no action were
taken?
Consideration of
all of the above will assist in making a
choice among alternative designs.
A sample
benefit/cost analysis is offered on the preceding
pages. It is intended to help project planners compare
alternatives
according to the four basic criteria: economic returns,
technical resources, social and cultural, considerations,
and
environmental concerns.
The alternative
designs are evaluated and measured for each
of the four criteria by using a simple scale numbered from 1
to
10. The lower end
(left) of the scale represents costs or negative
effects; the upper end (right) represents benefits or
positive
effects. The
five-point mark in the middle of the scale represents
a situation where benefits and costs are evenly
balanced. The
four ratings are then averaged to give a total average for
the
design. Alternative
designs can then be compared to select the
design that appears most beneficial.
There is no magic
about this measuring system. It is
relatively easy to use.
It allows alternatives to be reviewed:
Will
modifying parts of an alternative change its rating?
Development
workers will probably want to adapt the system to fit a
particular
situation
6 Implement project
Community
participation should be an integral part of
implementing a project.
Whenever possible, the use of local
materials and local technicians and craftspeople should be
encouraged. In this
way, future maintenance is not likely to be
beyond the community's resources.
Community pride, developed
through commitment to the project, successful participation
by
individual community members, and receipt of valued
benefits, is
the best guarantee for continued maintenance and long-term
benefits.
7 Monitor project
A plan to monitor
the project should be incorporated into the
original design.
This will allow the development worker and the
community to make any needed corrections in the project
design
and assist project implementation.
Furthermore, projects may
have environmental effects that must be monitored.
It is difficult
to predict all effects because environmental interactions
are
often more complex than anticipated.
For example, the changes
brought about by an energy project may not be immediately
apparent; the successful achievement of a project's energy
objectives may mask environmental degradation or other
negative
effects. Therefore,
it is important to continue to monitor the
project after it has been implemented.
A simple program of
measuring change can be set up to
identify trends that may be harmful First, it is necessary
to
collect and maintain relevant data for evaluating and
monitoring
the effects of a project.
For example, for a hydroelectric
project, it would be necessary to keep information on such
factors
as water quality, flooding, siltation, land displacement,
aquatic
life, etc. Such data
can then be used to help identify the maintenance
procedures necessary for the project's continued
operation. Unforseen
benefits may be encouraged, such as
improved health conditions from flood control measures.
Negative
trends may be corrected before the problems become too
severe, such as the planting of trees around the project
site that
cannot be used for fodder and whose planting would decrease
the
food available to livestock.
8 Evaluate project
Evaluating the
project provides information about what the
project achieved and, in particular, whether it met the
objectives
and needs initially established by the community and the
development
planner. These
evaluations also allow development workers
to share experiences with each other and provide much-needed
information on the activities of private voluntary agencies.
Examining,
analyzing, and reporting on the environmental,
technical, economic, social and other causes of success and
failure foster improved future planning and programming
decisions. This is
particularly important in a new field of work
such as energy development.
The special
character of the activities of private
development organizations requires complementary evaluation
techniques that are appropriate for projects involving the
poor.
These projects are usually low-cost, highly participatory,
innovative and place particular emphasis on process as well
as
quantitative results.
In tailoring an evaluation to fit your
particular circumstances, the Evaluation Sourcebook (Santo
Pietro, ed., 1982) could be very helpful.
VITA is a
repository for information that may be helpful for
your needs. Through
VITA you may make information on your
projects available to others.
Chapter VII
ENERGY
SOURCES AND ENVIRONMENTAL CONSIDERATIONS
The environmental
concerns associated with a variety of
small-scale energy sources are discussed here.
The points made
are intended only as guidelines, since specific environmental
benefits and costs depend largely on local conditions.
Although human
energy is not discussed directly in this
section, the substantial contribution of human energy has
been
stressed throughout this manual Specific energy technologies
may affect human health, use of time, and income as well as
cultural and behavioral patterns.
Perhaps the greatest challenge
is to find technologies that reduce the time needed to
complete a
task, maintain or increase income, and are adaptable to
socio-cultural norms.
Women's needs and chores are a special
case. The
nutritional and health status of people will directly
affect the amount of work they can accomplish.
Since natural
resource degradation reduces agricultural productivity and
therefore the amount of food available to fuel human energy,
the
uses of specific sources of energy should be carefully
evaluated in
terms of their impact throughout the agricultural resource
system.
Solar energy
The sun is the
ultimate source of clean and abundant energy.
For thousands of years it has been used directly by people
to dry
food and clothes, to warm homes and courtyards, or to
evaporate
water from salt ponds. <see image>
ese19x83.gif (437x437)
Indirectly, solar
energy makes the wind and water move.
Intercepted by green plants on land and sea, it becomes the
source
of energy for all life on earth.
This energy is released whenever
people burn wood, coal, or petroleum products.
Solar energy has
the potential for providing even more than
this. Converted to
electricity by photovoltaic cell, it can be
used to supply power to motors, refrigerators, lights,
communications equipment, and the like.
When concentrated or
"trapped," solar rays can generate high
temperatures for rapid
drying, cooking, and baking.
Most developing countries
lie in a belt between 30[degrees]N and 30[degrees]S
of the equator, where the average solar power is 700-800
watts
per square meter, or six kilowatt-hours per day with eight
hours
of sunshine. If it
were possible to capture even half of the energy
falling in one day on one square meter of surface, it would
be
sufficient to cook food for an entire family plus do the
work of
three adults.
However, the great
abundance and versatility of solar energy
carry certain limitations.
The most obvious is that solar energy is
directly available only during daylight hours when skies are
not
overcast. For use at
other times, the energy must be stored,
either in chemical form in batteries, or as retained heat in
water,
rocks, or other such materials.
Another limitation
of solar energy is that by the time it
reaches the earth, it is very diffuse and must be trapped or
concentrated.
Usually this can be done by using durable
transparent or highly reflective surfaces and a certain
amount of
space. Even with the
most efficient photoelectric cells it would
take over 10 square meters of collector surface to power a
small
water pump or grain mill.
If the energy is to be used for cooking
or baking, a minimum area of 1.5 square meters may be
required.
The use of solar
energy generally has no adverse impact on
the environment at the local community level.
To the extent that
solar devices may reduce the consumption of fossil fuels,
dung, or
fuelwood their use has measurable environmental benefits.
However, since
solar energy can be used in so many different
ways, it may be helpful to consider briefly some of its
possible
functions.
Drying:
Low-frequency heat radiation from the sun
passes
easily through a transparent window of a box.
Once inside,
however, the heat rays change and are unable to pass back
out of
the same window.
This is how solar heat energy is "captured."
A solar food dryer
is essentially a box with at least one
ese20x85.gif (437x437)
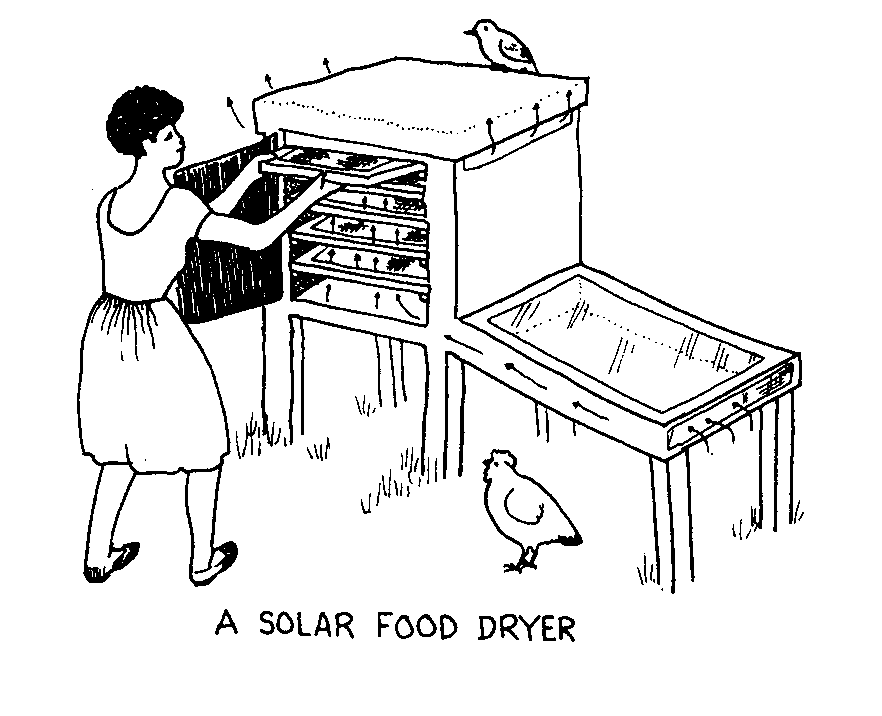
transparent side where solar energy raises the inside
temperature
and sets up a ventilating convection current of air.
Fruit, grain,
vegetables, and fish can be dried inside.
Food is traditionally
dried by exposing it directly to the sunlight in the open
air. A
solar food dryer will do the same job more rapidly, using
less
space, and with much less spoilage.
Moreover, there is less
interference from flies, birds, and other animals.
A solar dryer
requires a large amount of transparent glazing
material. Plastic
sheeting stretched over wooden frames is probably
the least expensive and most adaptable material.
However,
most plastics eventually lose much of their transparency and
become yellow and brittle under long exposure to the sun's
rays.
Glass does not yellow with age, of course, but it is often
very
expensive in poor countries.
Glass is also heavy and fragile. <see image>
ese21x86.gif (437x437)
Cooking:
At present, cooking with solar energy
appears
suitable only for food that can be baked or simmered for
long
periods without much attention.
Breads, beans, rice, many
sauces, and cereals may be adapted readily to solar
cooking. Most
disc reflector stoves (not solar ovens) require frequent
adjustment
of focus throughout the day.
Foods that require frying, stirring,
or other manipulation are difficult to prepare with solar
heat.
The use of solar
energy for cooking has not been widely
accepted by women in poor countries.
There are many reasons for
this:
*
Unwillingness to cook in the hot sun with
the bright glare
of a reflector.
*
Fear of the intense heat at the focal point,
which can
cause burns and
eye damage.
*
Restriction of cooking time to bright
daylight hours.
*
Stove designs that limit pot size and make
it awkward to
stir or
manipulate the pot contents.
*
Stoves that are unstable and easily damaged
by winds,
domestic
animals, and curious children.
*
Lack of replacement parts, repair skills,
and facilities.
*
Initial cost of solar appliances.
Electricity
generation: The technology for
converting solar
energy to electricity continues to make rapid progress.
Photovoltaic cells are now available with conversion
efficiencies
of 18 percent at a price that continues to decline.
Maintenance of a
photovoltaic system is limited to regular
cleaning of the panel surfaces.
However, the cleaning must be
carried out by trained individuals designated to maintain
the
system.
A National
Aeronautics and Space Administration (NASA)
pilot project in Upper Volta demonstrates the benefits of
photovoltaics to a rural village.
The system was installed in 1975
and later expanded.
Early technical and design problems have
been resolved, and the village now has a reliable source of
electricity. The use
of this energy is governed by a local
cooperative organization The power runs a grain mill, water
pump, small refrigerator, and (with rechargeable batteries)
a few
electric lights.
Income from the
mill is sufficient to acquire spare parts and
maintain the system.
One indirect benefit has been evening
reading instruction made possible by the electric lights.
The NASA project
was quite expensive, but, as a pilot
project, shows the potential for photovoltaics in a rural
setting
when they become more economically feasible.
However, rural
electrification through photovoltaics is still several
decades
away. The advantages
of simplicity and reliability must be
matched with further improvements in conversion efficiency,
a
longer functional life of the solar cells, and above all
reduced
costs.
According to one
source, there have been some negative
environmental effects of this project.
Due to the ease of lifting
water for animals, herders tended to remain in the village
for
longer periods. This
change in herding practices resulted in some
overgrazing. With
less fodder available around the village, the
raising of small animals by some women was negatively
affected.
More cattle damage to crops was also reported.
Because the
water system installed was a lifting rather than a delivery
system,
women spent as much time carrying water as before the system
was installed, but, with the new system, had to wait in line
behind
the herders.
Solar ponds:
A solar pond is a very large solar heat
collector
that operates on the same principle as the solar food dryer.
However, instead of trapping heat rays under a transparent
window, the heat is trapped under several layers of salt
water.
The pond has fresh water on the surf ace and very salty
water at
the bottom, with a salinity gradient in between.
This system can
generate heat to temperatures as high as
100[degrees], which is high enough to be used directly
(water heating, for
example). In some
parts of the Middle East the energy is often
used with a special engine (Rankine cycle) for pumping water
or
generating electricity.
Solar ponds can
create serious environmental damage; their
design and construction require assistance from those
skilled and
experienced in this technology.
Large amounts of salt are
required, and a leak in the bottom of the pond could
seriously
contaminate ground water supplies.
Also, the steeply sloping
sides could lead to accidental drownings of people and
animals.
Because of the high temperatures, objects sinking to the
bottom
cannot be easily retrieved without special equipment.
The hot
brine of a solar pond corrodes many metals.
Finally, water
evaporated from the pond surface must be replaced by water
from
other sources.
Wind
There is nothing
new about harnessing the energy of the
winds. Since ancient
times wind has been used for sailing boats,
lifting water, and threshing grain.
More recently, it has been used
to generate electricity.
Properly designed, maintained and
located to match specific tasks, wind machines can provide
years
of reasonably reliable service.
In developing
countries a water-pumping wind machine is
ese22x90.gif (437x437)
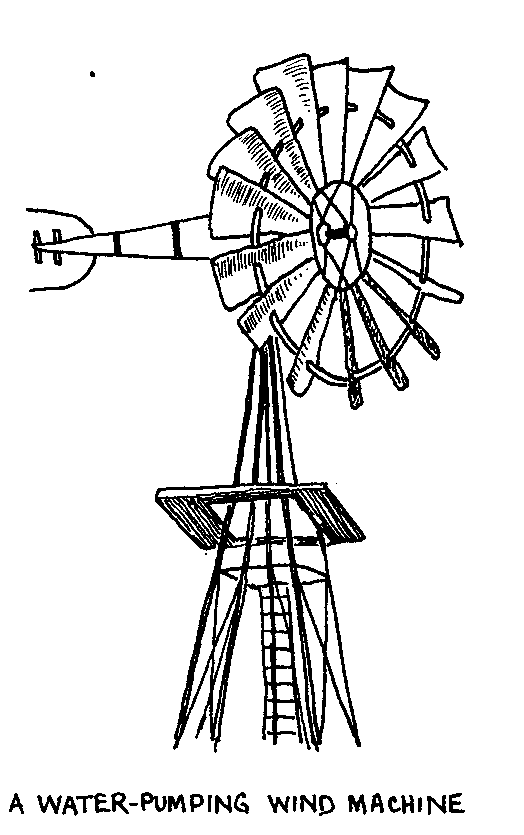
particularly suitable, both for irrigation pumping and for
supplying
potable water. When
water is pumped from the ground, the well
can be closed and protected from contamination.
On the
Philippine island of Higatangan, 1,600 people depend on
water
pumped by two wind machines, each with rotors three meters
in
diameter. In Africa,
several Malian fishing villages use wind
irrigation systems to increase yields in vegetable gardens,
providing a diversity of income and food supply sources.
One limitation to
any
wind machine is that it
runs only when the wind is
blowing. A steady
breeze
day after day is uncommon
in most parts of the world.
Before considering wind
power at a particular site,
it is important to know the
short- and long-term patterns
of local winds. A
wind-powered irrigation
system has little value if
the air is calm when water
is needed most. The
same
is true of grain mills and
any other wind-powered
device.
Compared to other
renewable energy systems, wind machines
have more moving parts, which are exposed to much stress,
not to
mention rain and dust.
Months of spinning and vibrating can
loosen important components or cause parts to become
worn. A
regular program of surveillance and maintenance is essential
to
keep the machine operating well.
Spare parts must be on hand,
along with someone who knows how to make necessary repairs.
The Third World is littered with the relics of wind machines
that
have failed simply for lack of replacement parts and
maintenance.
Certain precautions
are important to avoid possible
environmental effects from wind machines.
For example:
*
There is a danger with wind-driven water
pumps of
pumping more
water than is needed for irrigation,
livestock, or
domestic uses. This wastes water and
may
also create an
unhealthy situation around the pump. An
automatic
shut-off mechanism solves the problem.
Moreover, as
with any newly installed water system,
overgrazing near
the water supply can be a serious
problem.
*
In most cases, wind machines should be
mounted on a
tower at least
40 feet off the ground and 15-20 feet above
any nearby
obstruction, such as a building or tree.
This
makes the
mechanism highly visible, difficult to service,
and dangerous if
it topples. Mounting the machine on a
roof is likely
to cause vibration noise and apply unwanted
stress to the
roof.
*
The rotor must be equipped with an automatic
feathering
device to
protect the machine from winds exceeding its
design
capacity. There should also be
protection from
lightning
damage.
*
Vertical-axis machines generally require a
larger site than
comparably sized
horizontal-axis devices because of the
wider spread of
supporting guy wires.
*
When using lead-acid batteries for storing
excess
electricity, it
is important to keep them well ventilated
to avoid the
accumulation of explosive hydrogen and
oxygen gases.
Water (Hydropower)
Under certain conditions
it is possible to gain useful energy
from flowing water.
Hydropower for mechanical or electrical
energy is produced when the pressure of flowing water is
directed
at a waterwheel, turbine, or hydraulic ram.
Waterwheels, which
produce powerful mechanical energy at slow speeds, are best
suited for applications such as grinding grain or lifting
water.
Water used to produce electrical power is generally applied
at
high pressure to a specially-made turbine, which can be as
small
as 10 centimeters in diameter.
Hydraulic rams are essentially
automated water pumping devices that use the kinetic energy
of
water flowing in a pipe to lift the water higher than the
source.
Small rivers and
streams can provide the energy source for
small-scale applications.
Called micro- or mini-hydro, depending
on the amount of power generated, such applications function
either with or without a dam, depending on local
topography. The
most environmentally sound way to tap this resource is to
take
advantage of naturally occurring gradients that do not
require
construction of a dam.
This will also be the cheapest option.
It
requires a relatively steep stream gradient and good
year-round
flow.
No-dam hydropower
production requires diverting some water
ese23x93.gif (540x540)

from the stream and passing it through a channel to the
power
converting device.
This channel may be open, as in the case of
most water wheels, or it may be a closed pipe, which is
typical for
hydraulic turbines.
The channel does not slope downward as much
as the stream, so that after a short distance the water
level in the
channel is higher than that in the corresponding section of
stream.
This difference in height is called the
"head". The maximum
power to be derived from the water depends on the size of
the
head and on the maximum rate of flow through the channel
No-dam hydro projects have a minimum of environmental
drawbacks,
since they divert water flow along short sections of the
stream and do not flood the land.
In areas where
the stream flows gently and a long channel is
not practical, it is tempting to create a head over a short
distance
by constructing a dam across the stream.
This creates a reservoir
of water that may have many beneficial uses, such as for
irrigation.
However, dams
both large and small are widely viewed as
environmentally problematic.
They should be undertaken only
with skilled professional help.
Even with such assistance all the
problems will not be immediately apparent.
Some of the problems
that may be encountered include:
*
Inundation, or flooding, of the land behind
the dam may
cause loss of
plant and animal life, increase in soil erosion
around the
reservoir, reduced land available for food
production;
changes in water temperature that can affect
quality of the water.
*
Alteration of the normal flow of the stream
will reduce
availability
of nutrients and sediment downstream for
crops and for
fish life. It can also threaten fish
migrations and
hinder navigation.
*
Increased incidence of water-borne diseases
is a common
effect of the
creation of a large body of still water that
creates a
vector for disease.
*
Insufficient attention to geology and
topography of the
area may
result in a real threat to public safety as the
dam may not be
able to withstand the force of the moving
water.
A special note is
appropriate concerning the environmental
impact of hydraulic rams.
With few moving parts, hydraulic rams
are generally reliable and effective.
However, they are also very
noisy, sounding a loud "Clack!" every 1-2
seconds. This can be
extremely annoying to people living close by.
Biomass
The importance of
biomass (fuels derived from organic
materials such as trees, crop residue, and dung) as a staple
fuel in
developing countries can hardly be overstated.
More than 200
million people depend on wood to meet their basic energy
needs,
mostly for cooking and heating.
<see image> The only other reasonable, i.e.,
ese24x95.gif (437x437)
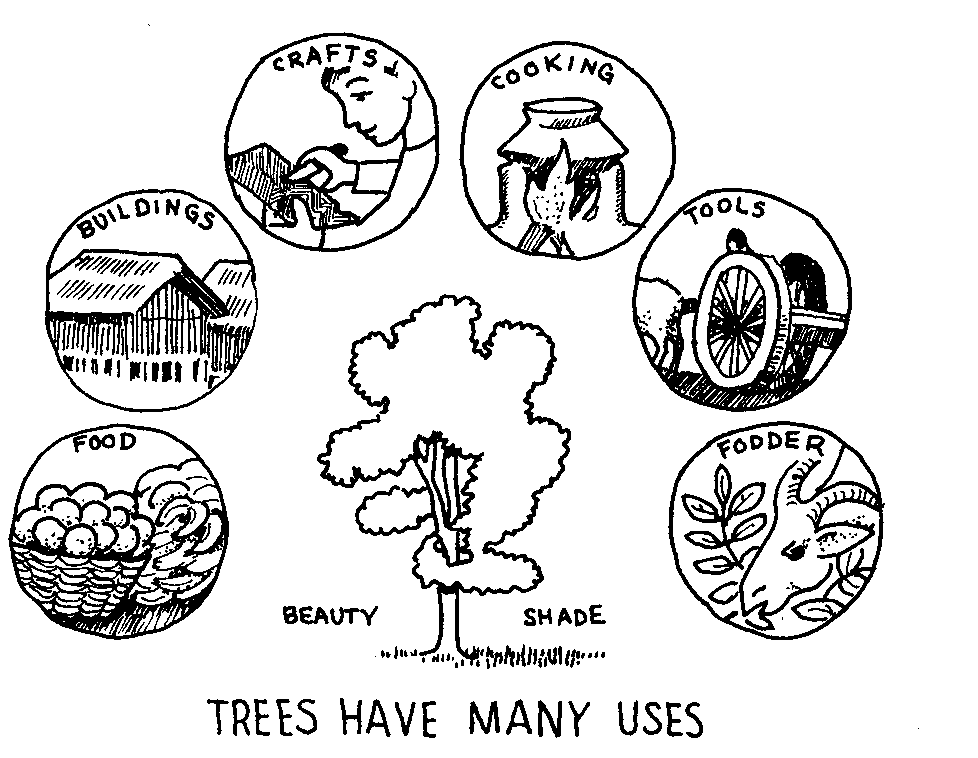
less costly, alternative for them is to burn animal dung,
straw, or
other agricultural wastes.
Fuelwood:
With the population of the Third World
increasing
by over three percent per year, the consumption of fuelwood
has
never been greater.
At the same time, overgrazing, heavy
timbering, climatic changes, and the expanding demands of
agriculture are rapidly destroying the world's remaining
forests.
Fuelwood, which in the past had always been considered
"renewable," is now being consumed as a finite resource.
The increasing
scarcity of fuelwood causes much hardship
among the poor. In
the capital cities of the Sahel, for example,
people often pay more for wood than for the food they
cook. In
rural areas the cost of wood is measured in the time and
effort it
takes to collect it.
For the most part, wood is seen as a public
resource that anyone may take, and yet no one is responsible
for
its replacement.
This is a familiar dilemma wherever the earth's
resources are involved.
Most people burn
wood by necessity rather then by choice.
While smoke from the fire may repel unwanted insects, it
also
irritates the eyes and damages the lungs.
It blackens pots,
utensils, and whole kitchen interiors.
The burning characteristics
of wood include distinct "flaming" and
"coaling" phases that
complicate attempts to use the heat efficiently.
These disadvantages
are made even worse whenever dry wood becomes damp.
The practice of
burning charcoal for domestic energy is often
seen as an unnecessary squandering of fuelwood.
Converting wood
to charcoal sacrifices as much as 80 percent of the original
energy. On the other
hand, where long distances are involved, it
may actually be more energy efficient to make and transport
charcoal than to haul the original quantity of wood.
Moreover,
when cooking, it is possible to use heat more efficiently
from
glowing charcoal than from a flaming wood fire.
So, whether it is
better to use charcoal or raw wood depends on at least three
factors: where the
fuel comes from, how it is transported, and
how it is used.
For many people
in rural areas, trees and shrubs have other
uses besides providing energy.
They are a source of fodder for
domestic animals, especially in dry seasons when grasses are
less
available. Often
leaves are a staple in local foods, or they are
important ingredients of medicinal teas and drugs.
Fibers for
basketry and cord, large fronds for roofing, and straight
poles for
construction are also derived from trees.
Trees and shrubs
play a dominant role in land-based
ecosystems in land-based ecosystems.
Their leaves and branches
shade the soil and cushion the impact of heavy rain.
Roots hold
the soil and help retain water.
Roots and leaves provide the soil
with important organic material and scarce minerals.
Decaying
organic material creates a favorable soil structure that
helps
absorb water and resist erosion.
Trees and shrubs can create
windbreaks, reducing wind velocity at ground level and
helping
retain soil moisture.
With widespread
deforestation these important functions are
ese25x97.gif (437x437)

lost. The changes
this brings vary according to local climate,
topography, and other factors.
In general, the results include an
increasingly harsh environment, with increased soil erosion,
degraded soils, silted waterways, and possibly lowered water
tables. Especially
alarming is the loss of soil fertility and reduced
food production.
One long-term
solution to deforestation is an intensive
program of forest management.
Many local species, when
properly cultivated, can develop sustained yields much
greater
than unmanaged forests.
Village woodlots and large-scale tree
plantations using fast-growing species are other possible
methods
of increasing wood supplies and maintaining the ecosystem.
Additional benefits of new trees may include forage for
domestic
animals, nectar for bees, nitrogen fixation for increasing
soil
fertility, and the full range of soil and water conservation
functions.
Information on sustainable forestry projects can be
found in Environmentally Sound Small-Scale Forestry Projects
by
Peter Ffolliott (published by Codel/VITA, 1983).
On a short-term
basis, much can be done to reduce the rate
of domestic fuelwood consumption.
Cooking over an open fire or
on a poorly designed stove can waste energy.
Reductions in
fuelwood consumption can be achieved in a number of ways:
*
Shield the open fire from drafts and breezes
so that the
flames will
lick the pot directly.
*
Protect fuelwood from moisture so that it burns
dry and
yields the
highest possible heat energy.
*
Cover all cooking pots with well-fitting
lids.
*
Arrange to have pots seated at the proper
distance from
the fuel bed
(that distance being roughly equivalent to
half the
maximum pot diameter).
*
Where possible regulate the draft if using a
stove.
*
Soak dry beans or grains overnight to reduce
cooking
time.
Even better, soak them in a tenderizing
solution,
such as that
derived from papaya fruit.
*
Use a haybox (an insulated, heat-retaining
box) to cook
foods
requiring long simmering. Or use a
haybox to keep
noon leftovers
hot so no reheating is needed in the
evening.
*
Extinguish the fire the moment the food is
cooked.
*
Take advantage of retained heat by cooking
over a simple,
enclosed stove
for warming water, drying wood, or
keeping food
hot after the fire is out. For more
discussion on
fuelwoods see Ffolliott, 1983.
Biogas:
Using plant and organic wastes to generate
clean,
combustible gas can be an attractive prospect in some
situations.
Biogas production can also yield a quality fertilizer and
soil
conditioner, which the Chinese report has boosted crop
production
as much as 130 percent.
In some areas biogas production has
reduced the incidence of hookworm and other parasites by
providing safe disposal of human feces. <see image>
Finally, the substitution
ese26x99.gif (540x540)
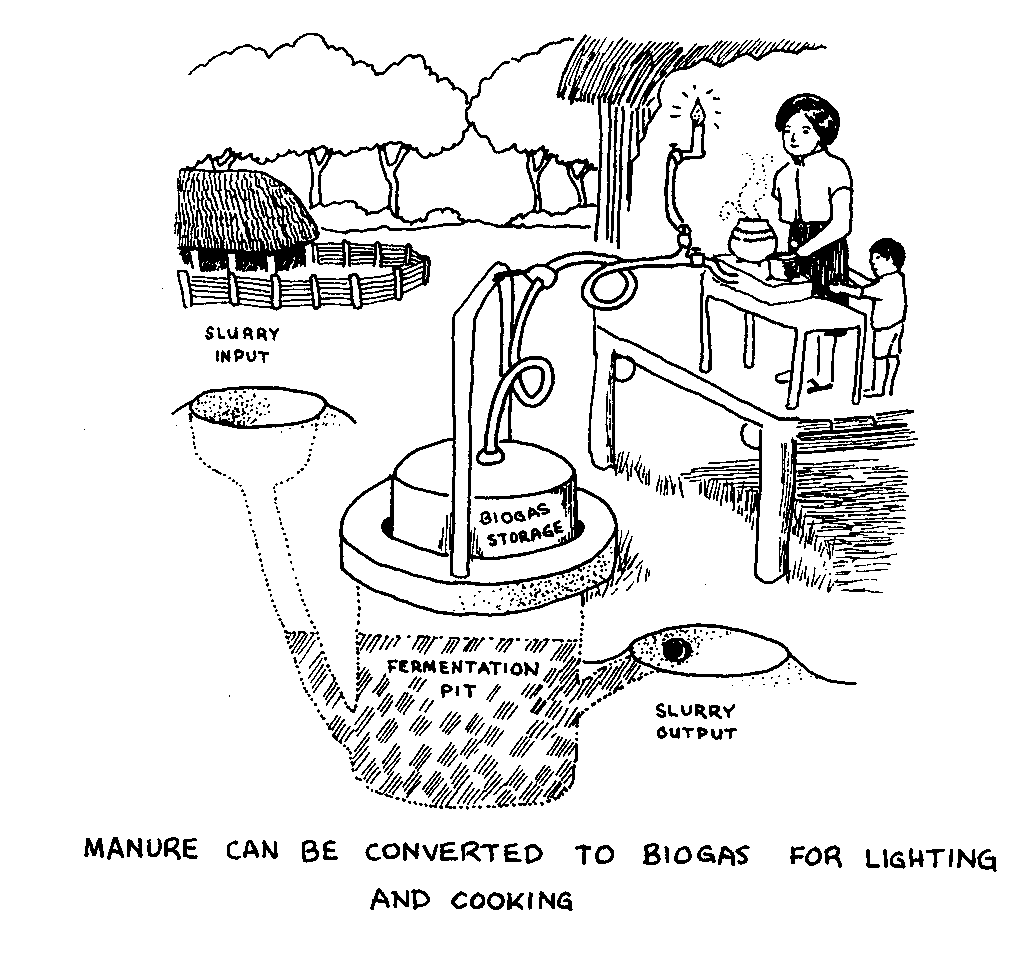
of biogas for wood or dung fuels may have other valuable
health
and environmental benefits.
Biogas is a
mixture of 60-70 percent methane plus carbon
dioxide, water, and often hydrogen sulfide gas.
One popular use is
for night-time lighting, where a bright lantern may consume
only
0.7 cubic meters (2.5 cubic feet) of gas per hour.
For cooking, a
single 5-10 centimeter (2-4 inch) burner consumes 0.2-0.4
cubic
meters (8-16 cubic feet) per hour.
Refrigeration consumes
slightly over one unit volume of biogas per unit volume of
refrigerated space per hour.
When substituted for diesel fuel,
biogas burns very cleanly, with 7 cubic meters providing the
energy equivalent of 4 liters of fuel (250 cubic feet per
gallon
fuel). In China, a
fuel of 70 percent biogas and 30 percent diesel
oil is said to provide power to some 150 small-scale
electrical
generators.
Like wind and
hydropower, biogas production is practical only
when certain conditions are met.
In addition to a proper digester,
there must be:
*
A steady, year-round supply of organic
material that
provides the proper balance of carbon and
nitrogeru Fresh
manure from
one cow can yield 0.17 cubic meters (6 cubic
feet) of gas
per day. The same amount of gas can be
generated from
the fecal wastes of nine adult people or
30 large
chickens.
*
An adequate supply of water sufficient for a
6:1 ratio
with dry
organic solids fed into the digester. A
biogas
unit using cow
manure, for example, initially requires at
least 3.5
liters of water for every 0.1 cubic meter of gas
produced (1
gallon per cubic foot). Once the
digester is
operating
effectively, much of the liquid overflow
(supernatant)
can be recycled in place of fresh water.
*
The daily services of a responsible person
knowledgeable
in digester
operation. There are two types of
digesters:
those able to
accept a small continuous flow of input and
those
requiring a single large quantity of material (batch
loaded).
A continuous--feed system requires
monitoring
digester
performance, preparing and adding raw
materials, and
disposing of the supernatant and sludge.
A
batch-loaded
digester requires less daily attention, but
demands much
labor whenever the batch is changed.
In China,
household and village biogas systems have been
built and used with some success.
However, recent reports
indicate a number of problems.
Experience may differ in regions
where water is scarce or where livestock roam freely and
distribute their manure around the countryside.
Often a biogas
generator is more effectively installed in an institutional
setting
than in a village or household.
In Africa, biogas operations have
been used at schools, hospitals, military installations, and
prisons.
Although biogas
digestion is widely regarded primarily as an
energy-producing technology, it can also play a major role
in
sewage disposal, agricultural production, fish farming, and
livestock maintenance.
It may be that biogas digestion has its
greatest potential in integrated applications where energy
production is but one part of a larger system.
Sludge from
biogas systems is rich in readily available plant
esex101.gif (486x486)
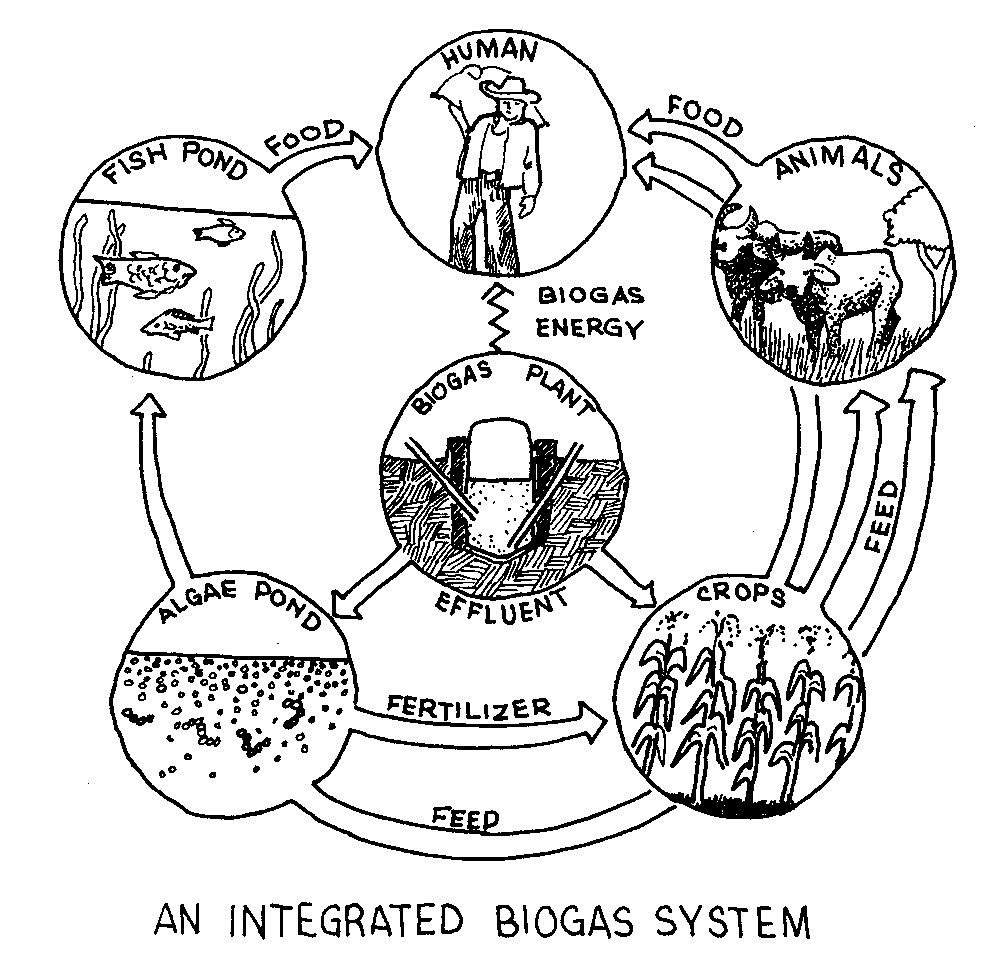
nutrients. Where
fish culture is feasible, a limited quantity of
sludge may be used to support algae and insects, which are
then
fed to the fish. A
more common use for digested sludge is to
improve soil fertility.
For maximum benefit, it is advisable to
mix sludge with the soil while it is still very fresh.
Sludge loses
much of its effectiveness when it stands.
If necessary, sludge
may be stored in a pit or large container and then covered
to
minimize exposure.
This will probably be necessary because
fertilizing is seasonal but sludge is produced continuously.
The operation of
a biogas digester presents several potential
environmental problems.
With proper planning and operation
these can be minimized:
*
Special precautions are required if human or
hog wastes
are to be
used. People and pigs share similar
fecal-borne
parasites and
pathogens, and although few of these
survive the
digestion process, more study is required on
the safety of
handling digested sludge. Some
authorities
warn against
applying sludge to soil where root and
vegetable crops are cultivated.
In any case, raw fecal
wastes should
always be considered extremely hazardous.
If the
digester is built close to lavatories or livestock
sheds, the
excrement may be deposited directly without
unnecessary handling.
*
Disposal of liquid overflow (supernatant)
from the
digester may
occasionally present a problem.
Normally
this liquid is
clear and odorless, and also has some value
as dissolved
fertilizer. If water is scarce, the
supernatant
may be
recycled into the digester with new organic
feedstock. Otherwise, it can be
used to water plants or
moisten
composting materials. With an
improperly
working
digester the supernatant may be dark and
extremely
offensive. If not recycled, this liquid
should
probably be
buried or mixed with soil in an isolated spot.
*
As with natural gas, precautions must be
taken to prevent
leaks of
biogas into the air. Surveillance is very
important,
since biogas normally is odorless and difficult
to
detect. In a closed room, leaking gas
can lead to
asphyxiation
or explosion.
*
In areas where manure or dung is considered
a free
community
resource, the installation of biogas digesters
may cause
unwanted changes in local economics. If
manure
suddenly becomes valuable it may become a
marketable
commodity, and will no longer be available to
the very
poor. The question of who stands to
lose or gain
from an energy
project is one that deserves attention in
the initial
planning phases.
Ethanol:
The production of ethanol (or ethyl alcohol)
is based
on small-scale technologies that have existed for centuries
for
making beers and spirits.
As a fuel, ethanol can be burned
directly in modified spark-ignition internal combustion
engines. It
may also be dehydrated and mixed with gasoline for a
high-octane
fuel. Ethanol is a valuable raw material in chemical and
pharmaceutical industries, so its production can foster a
profitable small industry.
Ethanol can be
made from a wide variety of plants containing
abundant sugars or starches.
Sugar cane, sweet sorghum, corn,
and cassava are most often used.
The plant material is crushed
or softened by soaking, fermented, and finally distilled to
isolate
the alcohol. The fermentation and distillation phases
require considerable
energy inputs, and it is debatable from an energy standpoint
whether the whole process results in a net gain or a net
loss.
Equally important
is the issue of using nutritious food to
manufacture a liquid fuel.
If energy crops are substituted for
food crops, the result could be higher food prices and less
available food. If,
however, ethanol is produced from surplus or
spoiling crops there is no competition with human food.
Also,
solid residues from ethanol production may be fed to
livestock as
a high-protein dietary supplement.
The disposal of
liquid residues, which may amount to 12-13
times the volume of the final product must be
considered. "Thin
stillage," as it is called, has a strong odor and high
acid content,
and contains may organic solids and solubles.
Land application of
thin stillage could be harmful to many kinds of soils, especially
those with high clay content.
Stillage should not be disposed of in
areas where it may flow into or contaminate lakes and
streams.
Finally,
significant amounts of water are used in the production
of ethanol. For
every unit volume of ethanol produced, about
16 volumes of water are needed for generating steam,
cooling,
and preparing mashes.
This demand for water must be evaluated
against available supplies and alternative uses.
Animal traction
Approximately 335
million draft animals provide about 150
million horsepower for at least 200 million people in two
thirds of
the world. This
source of energy is rarely given much attention,
but its contribution to economic activity, especially in
rural
areas, is very significant.
In various parts
of the world bullocks, oxen, buffaloes, horses,
camels, llamas, donkeys, and elephants are integral to
energy
systems supporting agriculture and transportation.
In agriculture
they are essential for plowing, harvesting, threshing, and
lifting
water. Animals
transport farm produce, other commodities, and
people. For short
distances with lengthy loading and unloading
times they are cost-competitive with trucks, and can often
travel
on terrain where trucks cannot. <see image>
esex105.gif (353x486)
Draft animals
would live longer and perform much better
with simple improvements in the design of carts and
harnesses.
Too often the harness pulls against the animal's neck
instead of
its shoulders. Not
only is this debilitating to the animal, but it
also prevents it from applying its full weight to the
task. Other
improvements include better herding, feed, and husbandry
practices.
Draft animals
need not compete with people for their food.
Usually they can stay healthy on a diet of natural
vegetation and
water. Environmental
problems can result from overgrazing. A
solution, if the animal is penned or tethered, is to provide
a daily
ration of water and fodder; this requires human energy to
bring
the water and fodder.
The use of animals that combine assistance
with farming and dairy products production is another
solution.
Chapter VIII
MATCHING ENERGY SOURCES WITH ENERGY USES
Energy is a means
to a specific end. It helps pump water,
cook meals, and plow soil. Not all forms of energy perform
these
tasks equally well. This chapter analyzes specific tasks
requiring
energy ("end uses") and discusses major factors in
selecting the
most appropriate way to provide energy for use in households
and
agriculture.
Because of the
enormous amount of time spent by people in
rural areas on survival as well as income-producing tasks,
the
effect of using specific energy technologies and sources on
time
and income, especially as it relates to the work of women,
should
be carefully considered.
Household energy
In households,
energy is used to prepare food, heat water,
provide space-heating and light, and carry out a variety of
other
tasks. In many countries it represents well over 90 percent
of all
energy used.
Cooking: Probably
no household task is performed as
regularly as cooking food. However, the energy requirements
of
cooking are as varied as the food itself. Cooking may
include
baking, frying, boiling, simmering, roasting, or steaming,
sometimes demanding high heat, sometimes low, or else one
followed by the other.
Perhaps the most
universal cooking task is the cooking of
rice, beans, or grains. Here water is brought to a boil, and
then
the mixture is simmered for up to several hours. Simmering
essentially means holding the mixture at a temperature close
to
boiling. Once that temperature is attained, little
additional
energy is needed beyond whatever is necessary to replace
heat
lost to the environment.
What sources of
energy are most appropriate for cooking
beans, grains, or rice? In a well-insulated box, solar
energy can
easily maintain the temperature of boiling water, although
bringing the mixture to its initial boil may take some time.
A
charcoal fire starts out relatively cool and gradually
builds up
heat, which is just the opposite of what is needed. Even
though
millions of women cook beans or rice over charcoal, they
usually
waste the excess heat produced during simmering. A properly
managed wood fire begins with hot flames licking the pot,
later
settling down to a bed of coals that produce a low, even
heat--and
this is exactly the energy pattern required. Greater control
of
the fire is possible with biogas, so the cook need use no
more
energy than is necessary for the task.
Actually, since little
or no additional energy is needed for the
simmer phase, a pot of boiling rice or beans may be removed
from
its source of energy and placed in a heavily insulated box
where
all the heat is trapped. This "haybox cooker"
concept has been
successfully used for hundreds of years in Europe, although
in
most developing countries acceptance of this idea has been
slow.
In many parts of
the world, the task of cooking does not make
the most efficient use of all available energy. Perhaps this
is
because energy efficiency is not the only factor important
for the
cook when selecting fuel. Besides a predominant concern with
cooking performance, other considerations in selecting fuel
may
include:
*
price or availability of the fuel
*
tendency of fuel to smoke excessively
*
convenience.
In the Gambia,
women who work in rice fields are interested
in cooking systems that work rapidly so that they can spend
as
little time as possible in the kitchen. In Burundi, many
women
have rejected smokey peat in favor of smokeless charcoal
even
though charcoal costs much more. In parts of western Niger,
the
women could burn twisted grasses or millet stalks, but they
prefer
smokey cow dung because the fire requires less attention.
The
needs and preferences vary widely, and yet they must be
considered when energy for cooking is being discussed.
Sometimes the women
in an area will use a fuel simply
because it is traditional. There has been no conscious
choice, and
the cooks may be unaware of the relative merits of any
alternatives.
Compared to almost
any other fuel, biogas for cooking is the
cleanest and easiest to control. Yet a number of problems
exist.
Collecting human and animal waste for the digester may be
impossible where there are social taboos against the
handling of
wastes. Families may not have enough livestock to provide
the
necessary amount of dung. With community biogas plants there
may be problems in the equitable distribution of the gas
among
community members. Also, there is the expense not only of
the
digester but also of the individual stoves or heating
elements to
replace the traditional system. Biogas systems require
training
for proper maintenance of the system.
Heating: In some
parts of the developing world, homes
require heat, at least during certain seasons. While not
always as
significant a problem as in the temperate regions, space
heating
can be an important need. It can often be met by the heat
produced from the cooking fire.
Most efficient
cookstoves enclose the fire and minimize the
transfer of heat to the surroundings. A stove specifically
designed for both cooking and body heating can be one
solution.
Otherwise, if a family adopts a fuel-efficient cookstove it
may be
obliged also to acquire additional energy for personal warmth.
In Korea, the
traditional "ondol" system is one that
successfully combines the functions of cooking and space
heating.
Unfortunately, it uses coal as a fuel, and the widespread
use of
this stove in Seoul is believed to contribute heavily to the
high
incidence of tuberculosis and other respiratory ailments and
carbon monoxide poisoning.
Lighting: For most
rural people night-time lighting is
provided by the moon, stars, or occasionally a flickering
wood fire
or kerosene lamp. However, women who cook after dark or
inside
a dark kitchen depend on light, often from the cooking fire.
If the
traditional fire is replaced by a fuel-efficient cookstove,
very
little light will escape and it will be necessary to find
other
sources of illumination.
Kerosene lamps (or
"paraffin lamps" in British English) are
widely used in urban areas where there' is no electricity.
However, the price of kerosene is very high and rising
steadily.
Biogas can produce
a very bright light when burned in a lamp
with a mantle. Electricity also gives very satisfactory
lighting.
Neither of these systems provides portable lighting,
however.
And both are costly.
While light is
often desirable in rural settings, it usually
carries no direct economic or survival benefits. For this
reason,
it may be best considered a possible side-benefit of energy
production where the primary use is more directly linked to
basic
needs and income generation.
Food processing:
Food processing includes husking, grinding,
oil extraction, pickling, drying, and refrigeration or
freezing.
These last two require significant amounts of energy.
Refrigeration and freezing, once begun, place a continuous
drain
on energy resources until the food is either consumed or
spoiled.
This makes it an expensive process, and in many areas
priority for
refrigeration will go to medicines rather then food. Energy
for
refrigeration can come from electricity generated in any
number
of ways. Biogas is also highly appropriate for
refrigeration.
For large-scale
drying of foods, a solar dryer can be
extremely practical. The drying is more even, more rapid,
than
most traditional methods, and the food is protected from
insects,
dogs, and other animals. See page 85 for further details on
solar
food drying.
Energy for agriculture
Energy is used in
all phases of agriculture. From land
clearing and management, to crop production, harvesting,
processing, and transport to market, considerable work is
required. In most areas of developing countries, much of the
energy for agriculture is from human labor, animal power,
and the
cycling of nutrients in natural biological processes.
A well-functioning
ecosystem is critical for dependable and
sustainable yields. Many heavily used energy sources play a
major
role in maintaining the well-being of the agricultural
ecosystem,
such as crop residues and dung gathered from fields, or
trees
planted around or near fields. In planning projects, the
competition between using these resources for energy and
using
them for their value in protecting soil and maintaining the
water
supply must be considered.
In some areas,
large amounts of energy are added to the
natural system (effectively changing the limiting factors)
to
increase yields. This includes chemical fertilizers,
pesticides, and
highly mechanized farming techniques. This can damage the
ecosystem, especially on marginal lands. Energy intensive
agriculture in such areas as the Sierra Madre of Mexico or
the
drought-prone plains of the Sahel can lead to serious
erosion and
other unwanted problems, making the land even less
productive
than before.
However, one should
take advantage of the impressive impact
that small infusions of well-placed energy for fueling
appropriate
technologies can have on crop yields. For example, if water
is the
limiting factor and is intermittent in supply, a
wind-powered
irrigation pump may be an answer.
Irrigation:
Irrigation is the application of water to crops to
increase their productivity. It may be used, for example, to
lengthen the growing season or to cultivate in arid regions
where
natural rainfall is insufficient. Under some circumstances,
irrigation can bring parasitic diseases and may ultimately
impair
soil fertility. While these issues are beyond the scope of
this
book, they should not be ignored.
Pumping water for
irrigation usually differs significantly
from pumping water for domestic use; this needs to be
considered
when seeking an appropriate technology. For example:
*
Water for irrigation is usually required in
larger volumes,
so the pumps are usually more powerful and
they operate
uninterrupted
for hours at a time.
*
Pumping for irrigation directly affects
agricultural
production and
hence income, while pumping domestic
water usually
has no direct financial benefits. Thus,
farmers may be
willing to invest more money or effort
into installing
irrigation systems. This is already evident
from the large
number of diesel-powered irrigation pumps
seen throughout
the Third World.
*
Irrigation pumping is usually not required
year-round. The
pumps may
actually be idle for months at a time.
*
Reliability in meeting the demand for water
is an
essential
feature of any irrigation system. It is wise to
have back-up
equipment and spare parts in case of
mechanical
breakdowns.
A range of energy
sources can be exploited to pump water for
irrigation. The best choice of technology depends, of
course, on
the specific circumstances, especially local farming
practices.
Here are a few guidelines:
Wind power can be
successful in pumping water. For
irrigation, it is
suitable only as long as there is a reliable
breeze at the right
time. You may construct a large tank
or above-ground
reservoir to store water for calm days, but
the evaporation
losses and high cost in this solution must
be carefully
weighed against possible benefits.
Electric pumps
operated by a photovoltaic system are worth
considering. There
is never any fuel to store or carry to the
pump site, which is
a great advantage if the system is far
from a town or
village. Because of the large initial cost of
equipment, a
photovoltaic system is probably most suitable
where there is a
long irrigation season with the likelihood of
a high profit from
the harvest. You should purchase only
equipment that has
been proven reliable in thorough field
testing.
Biogas may be used
in some instances, to run the motors of
irrigation pumps.
Alternatively, a mixture of 70 percent
biogas and 30
percent diesel fuel has been tried. It is
convenient to have
the sludge and supernatant produced close
to where they will
be applied to the soil. A possible
disadvantage is the
large size of digesters and great volumes
of raw materials
needed to provide adequate amounts of
biogas. A single
batch digester may be inadequate for even a
short irrigation
season, so either multiple batch digesters or
continuous feed
operations are required. Given the
importance of
reliability, biogas technology should be
considered for
irrigation only where it has already been
successfully used
locally for other functions.
Ethanol, diesel
fuel, gasifier engines, and other organic fuel
systems may all be
appropriate in specific situations. Here
the major issues
are fuel transport and storage, energy
efficiency, cost
effectiveness, and environmental impact. As
always, the
long-term effects must be considered, for they
are ultimately more
important than any short-term gains.
Animal traction is
sometimes well-suited for small irrigation
systems. Proven
schemes are available for using various
types of draft
animals to lift a continuous stream of water a
vertical distance
of 1-30 meters. The technology is relatively
simple and
reliable. When irrigation water is no longer
needed, the same
animals may be put to work transporting
the harvest,
cultivating the land, or performing other
functions. This
technology does require training and handling
animals, and the
availability of fodder in dry seasons.
Land preparation,
crop management, and harvesting: In
traditional western agriculture, these end uses depend on
farm
machinery such as tractors, plows, planting implements, and
threshers. Before such tools are adopted for small-scale
tropical
agriculture, you must be sure they are appropriate for local
conditions. Great erosion damage can result from plowing on
hilly
terrain where the soil structure is poor. Even on flat land,
farmers may find that a heavy rain can wash away soil to the
lowest depth of plowing. When using energy in agriculture,
much
environmental damage can be avoided by proper timing of all
activities and a wise selection of suitably scaled
machinery.
For small-scale
agriculture, animal traction still provides the
best low-cost energy in many situations. The animals must be
fed
and cared for, properly harnessed, and given only work that
does
not exceed their strength and endurance. The manure is an
added
benefit when properly applied to the soil or used in a
biogas
digester. If there is inadequate fodder, however, soil may
be
degraded by the animals eating the ground cover.
Another option is
the use of a hand tractor powered by
compressed biogas or any of the liquid fuels, such as
gasoline,
diesel, or ethanol. Small gasifier engines may soon be
proven
practical for hand tractors, although this will considerably
increase their weight. Also, there is some work currently
underway to develop dual-fuel engines to take advantage of
seasonal fuel availability.
There is increasing
interest in having energy to supply power
to machinery that comes directly from the land itself. This
entails the use of crop residues, such as rice husks or
animal
wastes, or the production of fuelwood or ethanol feedstock.
It is
possible to integrate the production of these energy
resources
with other uses of agricultural land. On the other hand,
growing
or using local resources to provide energy may actually
conflict
with food production.
Chapter IX
SUMMARY
There are no
cookbook recipes for successful energy projects;
different communities and local conditions require
adaptation in
approach and design of a project. However, there are basic
concepts and considerations that should be an integral part
of
planning and carrying out environmentally sound small-scale
energy projects. Below is a list of the
"ingredients" covered in
this manual:
*
Environmentally sound energy projects can
help maintain
a balance in
resource use, thereby contributing to the
regeneration
of resources. This can lead to long-term
availability
of renewable resources, the basis for
sustainable
energy development.
*
Energy is produced and used in different
ways. The local
ecosystem,
particularly those factors such as climate and
soil
fertility, affects the productivity of renewable
resources.
Socioeconomic structures and cultural values
affect a
community's choice of technologies for producing
energy and
the use the community will make of available
energy.
*
Traditionally women have played a key role
in the
collection
and use of energy sources in the Third World.
Energy
projects that ignore the knowledge and experience
of women may
increase rather than lessen the time and
effort
required to obtain energy from various sources.
*
The planning process requires information
about the
community
and data on the physical environment.
Socioeconomic information on the needs and use of energy
for families
and for different income groups helps
development
workers to predict better answers to the
following
questions:
-- How will
a proposed project affect the local
ecosystem?
-- How will
it affect various income groups involved in
the
project?
-- How can a
particular technology or new energy source
effectively be introduced to assure implementation?
-- How might
traditional attitudes and practices in
carrying
out the project affect the physical
environment?
Useful
information about the natural environment or local
ecosystem is
available from local people and sources.
Additional
technical information can be collected from
government
offices and other sources.
*
Community participation together with
guidelines that
include
environmental, social, cultural, economic and
technological considerations form the basis for decision
making and
reaching participant groups. Members of the
community
that will benefit from the project, especially
women,
should be involved in all levels of project
planning,
implementation and evaluation. Talking with
participants
is the best way to learn about local attitudes
and values,
community priorities, and other factors that
influence
energy use and acceptability of change and new
technologies.
*
The planning process helps explore present
problems and
avoid future
problems related to energy use and
production.
Examining ways to meet the energy needs of
a particular community involves
several factors:
--
Feasibility of developing additional energy sources or
improving
production of present sources or both
-- Benefits
and costs of developing new conversion
technologies
Improving
the efficiency of current energy "end uses"
(tasks/devices for which energy is needed, such as
improved
stoves where they are shown to be effective).
*
Planners can compare and measure various
energy sources
and end uses
by: collecting information as described
above;
considering multiple uses of an energy source; and
properly
testing the efficiency of end-use devices under
local
conditions.
*
Matching energy uses with the appropriate
energy sources
should be
based on environmental considerations that
minimize
negative effects on the availability and growth
of
resources.
Development
workers should find it useful to explore these
points further within the context of the local community and
the
specific environmental setting in which they are working.
Development workers of community-based organizations with an
established relationship with the people of the community
have a
special role to play in the area of sustainable development
projects. In these cases, implementing and monitoring
projects
that meet real needs is more likely. One further step that
would
be helpful is to share and exchange information about experiences
in the process of planning energy projects. Through talks,
workshops, and publications and other documentation, the
lessons
learned can benefit the work of other groups and
communities.
APPENDIX A
ENERGY CONVERSION TABLE
UNITS OF ENERGY
1 Kilocalorie (kcal) warms 1 kilogram (2.2 lbs) water
1[degrees]
Centigrade (1.8 F).
1 British Thermal Unit (Btu) warms 1 pound of water 1 degree
Fahrenheit.
1 foot-pound (ft-lb) lifts 1 pound 1 foot.
1 joule (J) lifts 1 kilogram 10.2 centimeters (4 in.).
1 kilowatt-hour (KWH) is energy used at the rate of 1000
watts
for one hour.
UNITS OF POWER
1 watt (W) = 1 joule per second
1 kilowatt (KW) = 1000 watts
1 Megawatt (MW) = 1000 kW
1 horsepower (hp) = 33,000 ft-lbs per minute
1 Quad - [10.sup.15] Btu (a million million Btu)
TO CONVERT
TO MULTIPLY BY
Btu's
cal 252
Btu's
ft-lbs 787
Btu's
joules 1055
Btu's
kWH 0.000293
cals.
ft-lbs 3.080
cals.
joules 4.184
kcals
Btu's 3.97
kcals
kWH 0.00116
ft-lbs
Btu's 0.0013
ft-lbs
joules 1.356
ft-lbs
kWH
0.000000377
ft-lbs
cals. 0.3247
joules
Btu's 0.0009
joules
cals 0.239
joules
ft-lbs 0.737
joules
kWH
.00000028
kWH
Btu's 3413
kWH
ft-lbs
2,631,000
kWH
joules
3,570,000
kWH
kcals. 859
horsepower
watts
746
horsepower
kcal/day 15,412
watts
horsepower 0.00134
watts
kcal/day 20.66
kcal/day
horsepower 0.000065
kcal/day
watts 0.048
APPENDIX B
ECOLOGICAL MINI GUIDELINES
FOR
SMALL-SCALE/COMMUNITY DEVELOPMENT PROJECTS
by
Fred R. Weber(*)
The following
short-form version of the CILSS/Club du Sahel
Ecologic Guidelines has been developed to meet the needs of
development workers at the community level. The original
version is available at cost ($5.00) from the CODEL
Environment
and Development Program. This paper was prepared by Fred R.
Weber as a result of discussions with private development
agencies at CODEL workshops on environment and development
in 1980.
The guidelines
assist in analysis of proposed activities and a
design that will minimize negative impacts. It is to be used
for
small-scale projects under $250,000. The general approach is
the
same as for the complete CILSS/Club du Sahel Ecologic
Guidelines. Methods
and procedure, however, have been
condensed in a form that is less time consuming and can be
carried out by project design personnel not formally trained
or
experienced in environmental analysis.
You are
encouraged to adapt the guidelines to your project.
CODEL welcomes comments on the usefulness of this tool and
reports on your experience in utilizing it.
(*) Fred R. Weber, a long time VITA Volunteer, is a forester
and
engineer who has worked for many years with private
development
agencies in West Africa. He is the author of many books,
including the classic resource Reforestation in Arid Lands
(VITA, 1977).
Introduction to the Guidelines
Begin with any
project in the community development area:
wells construction, school gardens, poultry raising, village
woodlots, access roads, and so forth. Any community activity
will, in one form or another, affect the environment
somehow.
Especially if "environment" is regarded in its
broadest form, not
only the physical aspects are affected but also health,
economics,
social, and cultural components.
The objective of
this exercise is to try to predict as far as
possible the various effects the proposed activity will have
in both
negative and positive terms. A project normally is designed
with
specific results in mind. An attempt is made to provide well
defined, "targeted" inputs to bring about some
improvement to
the people in the field. What is less clear is the nature
and extent
of incidental consequences these activities might bring
about that
are less desirable, in fact often adverse or negative.
In reality, more
often than not, the good will have to be
taken with some bad. Choices often involve trade-offs. The
trick
then consists of developing a system where these trade-offs
ultimately are as favorable as possible in terms of the
people
involved.
INSTRUCTIONS
In order to identify areas where possible adverse effects
may
occur, the basic question that should always be asked is:
HOW WILL PROPOSED
PROJECT ACTIVITIES AFFECT _____?
If we insert in
this question the components that together
make up the environment, we will get answers (and possible
warning flags) for those situations where otherwise negative
consequences "inadvertently" may result.
Explanation of Columns <see chart>
esex127.gif (600x600)
1. In the table on
Page 5, ask yourself the basic question for
each of the 18
lines (described below) and assign the
following values
in Column 3.
Very positive,
clear and decisive positive impact ....
+ 2
Some, but limited
positive impact .................... +
1
No effect, not
applicable, no impact .................
0
Some definite,
but limited negative impact ...........
- 1
Very specific or
extensive negative impact ........... -
2
2. A brief
explanation of the factors in columns 1 and 2:
Surface Water
runoff: peak and yields. How does the project
activity affect
runoff? How does it affect the peaks (flood
discharges)? How
does it affect the amount of water that
will flow
(yield)?
Groundwater: Its
quantity, recharge rates, etc. Also, does
the project alter
its chemical composition?
Vegetation:
Accent on natural vegetation. Will natural
cover be reduced
(bad) or increased (good)? How will natural
regeneration be
affected? Will there be additional (or fewer)
demands on trees,
bushes, grass, etc.?
Soils: Will the
project increase or drain soil fertility? Where
land surfaces are
affected by the project, is "optimal" land
use affected
favorably or adversely? Will erosion be more or
less likely?
Other: Basic
questions dealing with improvement or
deterioration of
factors such as wildlife, fisheries, natural
features. Also,
does the project follow some existing overall
natural resource
management plan?
Food: Will people
have more food and/or a more complete
diet?
Disease vectors:
A very important point and one that is
often overlooked:
Will the project create more standing
water? Will the
project increase (or create) fast flowing
water? How will
it affect existing water courses?
Population
density: How much will population density
increase as a
result of the activities? What contamination
conditions will
be altered? How? Will more health care
services be
required?
Other: Toxic
chemical, exposure to animal borne diseases,
etc.
Agricultural
productivity: Per capita food production
(staples or cash
crops), yields.
Volume of goods
or services: Will the project provide more
goods (food,
firewood, water, etc.) or less?
Common resources:
(Water, pasture, trees, etc.) Will the
project require
people to use more or less water, pastures,
etc.? Will it
eliminate any of these resources now available?
Will it restrict
access to these resources?
Project
equitability: How are benefits distributed? Who will
profit from these
activities? Special segments of the
population? How
"fairly" will the benefits be shared.
Government
services, administration: Will the project
demand more work,
"coverage" of government services? Will
it cause an
additional load on the administration: more
people, recurrent
costs, etc.?
Education and
training: How will it affect existing
education/training facilities? Strain or support? Or will it
provide
alternates? What about traditional learning (bush
schools, etc.)?
Community
Development: Will it encourage it, or will it
affect already
ongoing efforts? If so, is this good or bad?
Traditional land
use: Will it restrict existing use, harvesting,
grazing patterns?
Many projects promote "better" land use
but at the
(social) cost of some one or some group being
restricted from
using land, vegetation, water the way they
have been used
to.
Enerqy: How will
the project affect the demand for (or
supply of)
firewood? Will it increase dependence on fossil
fuels?
3. Column 4: This is
an arbitrary number based on experience.
4. Column 5: Choose
an adjustment factor between 1.0 and 5.0
depending on
whether a large number of people and/or large
areas are
affected. If a large segment of the population is
affected (say:
over 1,000 people) use a factor of 2.5. If
1,000 hectares or
more are involved, use 2.5 also. If both,
large numbers of
people and extensive area are affected,
combine the two:
use up to 5.0. Never use a factor less
than 1.0.
This step is
necessary because some activities may help a
handful of
people, but at the same time have some adverse
affect over large
areas. By assigning such area/people
factors to each
of the 18 lines, proper "weight" will be given
to these
conditions.
5. Compute the
adjusted score by multiplying columns 3, 4, and
5. Enter result
in column 6. Make sure to carry positive and
negative signs.
6. In Column 7: list
all impacts that are positive.
7. In Column 8: list
all impacts that are negative.
8. Now take another
look at Column 8. Here you'll find a
summary of the
negative aspects of your proposed activity.
Beginning with
the largest values (scores), determine what
measures you can
incorporate into your project, what
alternate
approaches can be followed to reduce these
negative values,
one by one. This may not always be possible,
but try to modify
your plans so that the sum of all negative
impacts will be
as small as possible. (Tabulate the new,
improved scores
in Column 10)
Modify, adjust, redesign your project so that the total of
all
"negative impacts" is as small as possible. This
is the essence of
"ecologically sound project design."
APPENDIX C
TROPICAL CLIMATES
There are three
principal types of tropical climates: the wet
or humid equatorial climate, the dry tropical climate, and
one
that is alternately wet and dry. <see map>
esex128.gif (600x600)
Wet or humid
equatorial climate is found in a band of approximately
5 degrees north and south of the equator.
It is characterized
by heavy rainfall (75-120 inches of rain per year), constant
heat and high humidity.
This includes the Amazon and Congo
Basins; West Africa south of the Sahel; parts of Kenya,
Tanzania,
and Madagascar; Malaysia; Indonesia; Papua New Guinea; and
many of the Pacific Islands.
Dry tropical
climates occur in two "belts" approximately
15-30 degrees north and south of the Tropics of Cancer and
Capricorn, which are characterized by hot arid weather and
deserts. This is
true of most of North Africa, Saudi Arabia, Iran
and Pakistan, and parts of Australia, Peru and Chile.
Climates that
alternate between wet and dry seasons are
found in between the wet equatorial band and the dry
tropical
belts. These areas
are found in south and southeast Asia, Africa,
the grassy plains of Venezuela, and eastern Brazil.
The length of
the rainy season and the amount of rainfall vary
considerably
among these areas and also yearly in a given area.
Rainfall
A major problem
in the tropics generally is the amount of
rainfall: there is often too much or too little rainfall.
Heavy
rains, especially in steep areas, crush soil structure, seal
off
underlying soil from the air, leach out necessary soil
nutrients
(wash them away) or push them too far into the ground for
plant
roots to reach them.
To assess
rainfall, one should take into account the total
amount of rain per year, and the variability and intensity
of the
rainfall.
Variability indicates whether sufficient water will be
available to generate power when it is needed, or whether
the
seasonal demand for crop residues could be met.
For instance,
even though the total annual rainfall in Santo Domingo,
Dominican Republic, is about the same as Katmandu, Nepal
(1400
millimeters per year), the rain in Katmandu is far more
concentrated
in certain months.
Soil Erosion
The rate of soil
erosion also differs between regions, due to
the amount and intensity of rainfall, the type of soil and
the
steepness of the area.
Soils in the tropics are generally less
fertile than in moist, temperate areas because they contain
less
organic material (humus) in which nutrients are stored.
These
soils can less afford to lose organic material from harsh
rains.
When vegetative cover is removed, bare, exposed soil rises
in
temperature hastening the oxidation and disappearance of
humus.
Shifting agriculture is a major means by which farmers in
the
humid tropics maintain crop production: as poor soils are
worn
out, they move to other areas.
Some exceptions are found in alluvial and
volcanic soils, and
in forest soils of tropical mountains that escape the
greater heat
of low altitudes and may be rich in humus.
The steep rivers in
these mountains carry rich alluvial soil from other areas that
enriches the farmland.
The same is true in parts of Uganda and
the Sudan. Volcanic
soil is found in various parts of the world.
Insolation and Wind
Insolation varies
regionally and seasonally. The angle of
the
sun varies in the regions away from the equator, and the
amount
of effective sunlight available depends on cloud cover.
This may
be quite important if the need for solar energy coincides
with the
rainy season.
In some places,
relatively infrequent wind gusts require
windmills that can withstand a wide range of wind
speeds. In
other areas, such as some islands in the Caribbean,
windmills must
be able to turn under a relatively slow but constant wind.
All these
features together contribute to an ecosystem that
is relatively fragile.
The risk of long-term damage from any large
project may be lessened through careful planning and
subsequent
monitoring.
APPENDIX D
BIBLIOGRAPHY
Addresses for obtaining these publications are listed in
Appendix
E, Sources of Information.
Arnold, J.E.M. "Fuelwood and Charcoal in Developing
Countries."
Unasylva, Vol.
29, No. 118. 1979.
Bassan, Elizabeth (Ed.). Global Energy in Transition:
Environmental
Aspects of New and Renewable Sources
for Development.
New York: UNIPUB. 1981.
Briscoe, John. The Political Economy of Energy Use in Rural
Bangladesh.
Monograph. Environmental Systems Program,
Harvard
University. 1979.
Cecelski, Elizabeth, et.al. Household Energy and the Poor
in the Third
World. Washington, D.C.: Resources for
the Future. 1979.
Chatterji, Manas (Ed.). Energy and Environment in the
Developing
Countries. New York: John Wiley
and Sons. 1981.
Darrow, Ken, et al. Appropriate Technology Sourcebook
Vols. I and II.
San Francisco: Volunteers in Asia. 1981.
deLucia, Russell J., Henry D. Jacoby, et al. Energy Planning
for Developing
Countries: A Study of Bangladesh.
Baltimore: Johns
Hopkins University Press. 1982.
East West Center. Rural Energy to Meet Development Needs:
Issues and
Methods. Boulder, Colorado: Westview Press.
1983.
El-Hinnawi, Essam. The Environmental Impacts of Production
and Use of
Energy. Dublin: Tycooly Press. 1981.
Environment Liaison Centre. Address List of Non-Governmental
Organizations
Working in the Field of Energy. Nairobi:
ELC. 1981.
Evans, Ianto and Michael Boutette. Lorena Stoves: Designing
and Testing
Wood-Conserving Cookstoves. Stanford,
California:
Volunteers in Asia. 1981.
Ffolliott, Peter F. and John L. Thames. Environmentally
Sound
Small-Scale
Forestry Projects: Guidelines for Planning.
New York: CODEL.
1983.
French, David. The Economics of Renewable Energy Systems
for Developing
Countries. Washington, D.C.:
U.S. Agency for
International Development. 1979.
Holland, R. et al. "Community Load Determination,
Survey
and System
Planning", in Small Hydro-Electric Powers.
National Rural
Electric Cooperative Association.
Hoskins, Marilyn with Fred R. Weber. Household Level
Appropriate
Technology For Women. Washington, D.C.:
U.S. Agency for
International Development. 1981.
Hoskins, Marilyn. Women in Forestry for Local Community
Development: A
Programming Guide. Office of Women
in Development, USAID, Washington, D.C. 1979.
Kamarck, Andrew M. The Tropics and Economic Development.
Washington, D.C.:
The World Bank. 1976.
Lichtman, Robert. Biogas Systems in India. Arlington,
Virginia:
Volunteers in
Technical Assistance. 1983.
National Academy of Sciences. Diffusion of Biomass Energy
Technologies in
Developing Countries. Washington, D.C.:
National Academy
Press. 1982.
National Academy of Sciences. (two parts). Energy for Rural
Development:
Renewable Resources and Alternative
Technologies for
Developing Countries. Washington, D.C.:
National Academy
Press. 1976 and 1981.
National Academy of Sciences. Firewood Crops: Shrub and Tree
Species for
Energy Production. Washington, D.C.:
National Academy
Press. 1980.
National Academy of Sciences. Methane Generation from
Human, Animal and
Agricultural Wastes. Washington, D.C.:
National Academy
Press. 1977.
Odum, Eugene P. Ecology. New York: Holt, Rinehart and
Winston. 1975.
Santo Pietro, Daniel (Ed.). An Evaluation Sourcebook for
PVOs.
New York:
American Council of Voluntary Agencies
for Foreign
Service. 1983.
Sivard, Ruth L. World Energy Survey. Virginia: World
Priorities. 1981.
Smil Vaclav and William E. Knowland. Energy in the
Developing
World: The Real
Energy Crisis. Oxford: Oxford University
Press. 1989.
Tinker, Irene. Women, Energy and Development.
Washington, D.C.:
Equity Policy Center. 1982.
Van Buren, Ariane QQ. A Chinese Biogas Manual. London.
Intermediate Technology Publications Ltd.
1979.
VITA/ITDG. Wood Conserving Cook Stoves: A Design Guide.
Arlington,
Virginia: Volunteers in Technical
Assistance. 1980.
NOTE: The Intermediate Technology Development Group,
Volunteers in Asia, and VITA publish many how-to books for
contructing specific technologies including solar cookers,
solar
stills, biogasplants, cookstoves, windmills, waterwheels,
hydraulic
rams, and dams. For addresses see Appendix E, Sources of
Information.
APPENDIX E
SOURCES OF INFORMATION
References can be obtained from:
American Council of Voluntary Agencies for Foreign Service
200 Park Avenue South
New York, NY 10003
USA
CODEL
79 Madison Avenue
New York, NY 10016 - 7870
USA
Environment Liaison Centre
P.O. Box 72461
Nairobi, Kenya
Equity Policy Center
2001 S Street, N.W., #420
Washington, DC 20009
USA
Harvard University
Environmental Systems Program
Cambridge, Massachusetts
USA
Holt Reinhart and Winston
521 5th Avenue
New York, NY 10175
USA
Intermediate Technology Development Group
9 King Street
London WC2E 8HN
United Kingdom
Johns Hopkins University Press
Baltimore, Maryland 21218
USA
John Wiley and Sons, Inc.
605 Third Avenue
New York, NY 10016
USA
National Academy of Sciences
2101 Constitution Avenue, N.W.
Washington, DC 20418
USA
Oxford University Press
Walton Street
Oxford OX2 60P England
Resources for the Future
1755 Massachusetts Avenue NW
Washington, DC 20
USA
Tycooly International Publishing, Ltd.
6 Crofton Terrace
Dun Laoghaire
County Dublin, Ireland
Dublin, Ireland
U.S. Agency f or International Development
Washington, DC 20523
USA
VITA
1600 Wilson Boulevard, Suite 500
Arlington, Virgnia 22209 USA
Tel: 703/276-1800 . Fax: 703/243-1865
Internet: pr-info[at]vita.org
Volunteers in Asia
Box 4543
Stanford, California 94305
USA
UNIPUB
345 Park Avenue South
New York, NY 10010
USA
Westview Press
5500 Central Avenue
Boulder, Colorado 80301
USA
The World Bank
1818 H Street, N.W.
Washington, DC 20433
USA
World Priorities
Box 1003
Leesburg, Virginia 22075
USA
BIOGRAPHICAL NOTES
Elizabeth A. Bassan, Author
During the preparation of this manual, Elizabeth Bassan was
working with the Sierra Club International Earth Care Center
in
New York City. Following that post, she joined the staff of
the
American Council of Voluntary Agencies in Foreign Service in
1982. Ms. Bassan is currently in Nairobi, Kenya on free
lance
consultancies.
Ms. Bassan took her training in International Affairs at
Columbia
University. Her experience includes paralegal work,
organizing
and participating in international conferences involving
private
development groups, and editing conference publications,
notably,
Global Energy in Transition: Environmental Aspects of New
and
Renewable Sources for Development (UN Conference on New and
Renewable 5ources of Energy, 1981)
Timothy S. Wood, Ph D, Technical Editor
Timothy Wood recently returned from two years in West Africa
as
Technical Coordinator of the Sahel Regional Improved
Woodstoves
Program with CILSS/VITA. He is currently Director of the
Environmental Studies Program and Associate Professor of
Biological Sciences at Wright State University in Dayton,
Ohio.
Dr. Wood was trained in biology and ecology at University of
Colorado in Boulder. His professional interests concentrate
on
controlled combustion of biomass for efficient production of
useful heat energy, environmental impact of development projects
in economically disadvantaged regions of the world, and
sound
technological solutions to environmental problems in these
areas.
Dr. Wood has contributed his services to the CODEL
Environment
and Development Program since 1980 when he served as a
resource person f or a CODEL t raining workshop at Lake
Mohonk,
N.Y. He remains closely associated with VITA as a VITA
Volunteer
and consultant in his field.
========================================
========================================