TECHNICAL PAPER #72
UNDERSTANDING SOIL EROSION
AND ITS CONTROL
By
Jim Chamberlain
Technical Reviewers
Robert S. Jonas
Fred R. Weber
Illustrated By
Frederick J. Holman
Published By
VITA
1600 Wilson Boulevard, Suite 500
Arlington, Virginia 22209 USA
Tel: 703/276-1800 * Fax:
703/243-1865
Internet: pr-info@vita.org
Understanding Soil Erosion and Its Control
ISBN: 0-86619-315-4
[C]
1990, Volunteers in Technical Assistance
PREFACE
This paper is one of a series published by Volunteers in
Technical
Assistance to provide an introduction to specific
state-of-the-art
technologies of interest to people in developing countries.
The papers are intended to be used as guidelines to help
people choose technologies that are suitable to their
situations.
They are not intended to provide construction or
implementation
details. People are
urged to contact VITA or a similar organization
for further information and technical assistance if they
find that a particular technology seems to meet their needs.
The papers in the series were written, reviewed, and
illustrated
almost entirely by VITA Volunteer technical experts on a
purely
voluntary basis.
Some 500 volunteers were involved in the production
of the first 100 titles issued, contribution approximately
5,000 hours of their time.
VITA staff included Patrice Matthews
and Suzanne Brooks handling typesetting and layout, and
Margaret
Crouch as senior editor and project manager.
VITA Volunteer Dr.
R.R. Ronkin, retired from the National Science Foundation,
lent
his invaluable perspective to the compilation of technical
reviews,
conversations with contributing writers, editing, and in a
variety of other ways.
Jim Chamberlain, the author of this paper, is a program
officer
for the Nitrogen Fixing Tree Association in Hawaii.
A specialist
in tropical forestry, he has experience in the Philippines
and
elsewhere in East Asia.
Technical reviewer Robert S. Jonas is a
soil scientist retired from over 30 years with the U.S.
Department
of Agriculture's Soil Conservation Service.
Fred Weber, the
other technical reviewer, is the author of Reforestation in
Arid
Lands (VITA, 1986) and a community forestry expert with
extensive
experience in Africa.
All three are active VITA Volunteers.
VITA is a private, nonprofit organization that supports
people
working on technical problems in developing countries.
VITA offers
information and assistance aimed at helping individuals and
groups to select and implement technologies appropriate to
their
situations. VITA
maintains an international Inquiry Service, a
specialized documentation center, and a computerized roster
of
volunteer technical consultants; manages long-term field
projects;
and publishes a variety of technical manuals and papers.
UNDERSTANDING SOIL EROSION
AND ITS CONTROL
by
VITA Volunteer Jim Chamberlain
1. EROSION AND SOIL
LOSS
Geological erosion is a natural, continuous process that
occurs
almost anywhere that water flows on the land.
It can also result
from the action of wind, changes in temperature, and the
activities
of living things.
Wind dislodges and moves soil particles.
Rapid temperature variation between day and night, not a
major
problem in most tropical climates, affects soil surface
structure.
Biological agents are lichens, mosses, and animals,
including
livestock that compact soils and overgraze vegetation cover.
Erosion by water receives the most attention in this paper.
Erosion forms many kinds of soil from rock and is controlled
by
such factors as rock properties, topography, vegetation, and
climate.
Some forms of erosion result in topsoil removal, rock
failures,
landslides, slumps, and riverbank cutting.
Erosion is usually accelerated by such human activities as
forest
destruction, traditional agriculture, grazing, construction,
and
mining. Whenever
vegetation is removed, as when forests are
cleared for agriculture, and the ground is exposed to
rainfall,
soil erosion by water and wind may increase.
On sloping land it
far exceeds the rate under natural conditions.
Accelerated erosion,
widespread throughout the tropics, is one of the most
serious
environmental and socioeconomic problems affecting rural
people.
Soil loss is affected by soil composition, type of cover,
soil
management practices, and microclimate conditions.
With highly
fertile soils erosion has little adverse effect on
productivity
but increases production costs.
In soils with medium rooting
depth and surface thickness, the effects of erosion can be
hidden
by the use of technologies that work these potentially
fragile
soils. Erosion of
marginal soils with shallow rooting depth,
found throughout poor countries, results in continued
decline of
crop yields.
Mismanagement of marginal soils can lead to permanent
loss of soil fertility.
Loss of a few centimeters of topsoil can reduce the
productivity
of good soils by 40 percent and poor soils by 60
percent. In the
United States, wind erosion over 30 years caused a loss of
30 cm
of topsoil, resulting in a 70 percent decline in wheat
yield.
Land-use planning should aim for an acceptable income and a
minimal
soil loss. Planning
for erosion control must consider these
factors: soil type,
extent of erosion, topography, location of
waterways and drainage, runoff diversions, size and
arrangements
of fields, cropping system, and tillage methods.
Vegetation is an
especially important tool for erosion control.
2. TYPES OF WATER
EROSION
It is important to recognize the kinds of erosion, because
each
type may require a different approach to its control.
The flow of water over sloping land may be the most erosive
factor
affecting soils.
Soil particles are dislodged or break from
the soil mass, disrupting the physical and chemical bonding
of
soils. Soil erosion
by water includes detachment, transport and
deposition of soil by raindrops and runoff.
Suspended soil particles
dislodge other lighter particles through abrasion.
The extent of erosion depends on the amount, velocity, and
turbulence
of the runoff. The
type of abrasive material being transported
also affects the extent, which also depends on the energy
of flowing water and amount of suspended material.
Velocity increases
as depth of flow and slope increase.
Turbulence increases
in proportion to the intensity of rainfall.
The major forms of erosion affecting agricultural lands are
sheet, rill, and gully.
Sheet erosion is caused by the even flow
of water over sloped lands.
It removes lighter soil particles,
organic matter, and soluble nutrients.
Its effects are less apparent
than those of other forms, but they can seriously affect
soil fertility and farm productivity.
Rill erosion occurs on sloped land dissected by small
parallel
channels running downhill.
If these do not interfere with normal
tillage practices they are called rills.
Soils that are easily
worked are more apt to form rills, and rills typically flow
together
and form gullies.
Two types of gully erosion create problems on agricultural
lands.
They are named for their distinctive cross-sections:
V-gullies
are identified by downward cutting centers, whereas the flat
bottoms of U-gullies are parallel to the slope of the
field. Control
measures for the two types are different, as described at
the end of Section 3.
3. AGRONOMIC CONTROL
OF WATER EROSION
Tillage Practices
Intense cultivation and harrowing break down heavier
textured
soils into easily transportable particles.
Changing the physical
structure of soils through tillage thus can make them more
susceptible
to erosion.
Conservation tillage, the practice of leaving
crop residue on the soil surface, can reduce sheet and rill
erosion as much as 90 percent.
One type of conservation tillage, called no-till, zero-till,
or
low-till, eliminates all plowing, disking, and
cultivating. The
new crop is seeded directly into the crop residue of the
previous
season. The system
conserves soil moisture, decreases runoff,
reduces soil loss and helps maintain organic matter.
In a research
study in Nigeria, zero-till prevented 96 percent of runoff
and 99.5 percent of soil loss on 10 percent slopes.
Unfortunately,
this strict variety of conservation tillage requires special
equipment (for instance, to loosen the soil under the crop
residue without turning it over) and expensive herbicides.
Contour Cultivation
In India, contour cultivation on 2 percent slopes reduced
soil
loss by 28 percent and runoff by 61 percent, compared to
traditional,
up-and-down plowing.
It is most effective on 3 percent to
8 percent slopes. On
steeper slopes, runoff may concentrate in
the furrows and if it breaks through may cause serious
erosion.
Contour cultivation on steep slopes must be supplemented by
other
methods.
Vegetation Cover
Well planned and managed vegetation cover can effectively
control
soil movement.
Vegetation protects soil against erosion by reducing
water movement and building soil structure.
It also affects
the soil surface, where running water does the most
damage. Vegetation
protects soils against erosion in a number of ways.
First,
it decreases the amount of rain reaching the soil by
intercepting
rainfall; the decrease was about 12 percent under forest
canopy
in a project in Indonesia.
The decrease, of course, varies with
the types of trees and management practices.
Second, leaves break
the initial erosive power of rain.
(However, they may also increase
the erosive power if drops concentrate and fall from
greater heights.)
Third, vegetation prevents the direct impact of
rain on the soil, which reduces soil compaction and clogging
of
soil pores. Fourth,
the increased formation of humus by vegetation
improves soil permeability and structure, improving its
capacity to retain moisture.
During natural fallow periods erosion rates tend to drop due
to
the formation of a layer of plant litter, invasion of weeds,
and
buildup of humus and organic matter.
In planted fallow areas, on
the other hand, erosion rates may increase.
For example, in
densely planted tree fallows, litter decomposes rapidly,
natural
ground covers will not grow due to excessive shade, and
water
flows freely over the land.
Removing the litter layer from under trees may increase
erosion
from 10 percent to 100 percent.
But removal of the canopy without
disturbing the litter layer affects the erosion rate by only
about 0.3 percent.
A complete soil cover protects against erosion except on the
steepest slopes. The
most effective vegetation cover to control
erosion is a multi-layered canopy of trees, shrubs, and
ground
cover. Multiple
layers slow the impact of raindrops, increase
rain flow over the stems, and increase the litter
buildup. Where
field crops are grown, cover cropping and intercropping help
to
control erosion.
Mulching
Mulching covers the soil with materials that reduce soil
moisture
evaporation and inhibit weed growth.
Mulching slows rainfall
infiltration and protects the soil from direct impact from
rain.
Mulches applied before the beginning of the rainy season can
reduce soil erosion and runoff.
Further, they build soil structure
and protect soil from extremes of temperature.
A study in Nigeria showed 50 percent more soil lost from
land
with no mulch than from land with a mulch layer of 2
t/ha. A 5-cm
layer of straw mulch almost eliminated erosion of bare
soil. In
any location, mulching is likely to control erosion and
bring
other benefits.
The best mulching materials have a high humus content, along
with
good infiltration rates and water storage capacity.
The properties
to look for in selecting mulches are listed below:
o
Withstand the forces of runoff; stay in
place
o
Last for several seasons; slowly decomposing
o
Allow water to percolate into the soil
o
Ease of application
o
Inexpensive; require low maintenance
Crop residues are an excellent local source for mulches,
particularly
if they are not required for other purposes, such as animal
feed, fuel, and roofing materials.
If limited supply and high
costs are not a problem, crop residues should be tried.
In addition
to their ability to aid in erosion control, they add humus
to the soil.
Cropping Patterns
Changes in the cropping pattern that will help reduce soil
movement
include intercropping, alley farming, use of grass strips,
and pasture improvement.
For example, conversion of cultivated
land to grassland can reduce erosion by at least 10 percent.
Producing feed crops for livestock presents an opportunity
to
integrate animal husbandry and erosion control.
Producing sufficient
fodder grasses reduces the need to graze animals; cutting
and carrying feed may reduce the space needed by the animals
and
allow for more crop land.
Alternating strips of protected plants
(vegetables) with protective plants (fodder grasses) will
trap
suspended particles and reduce soil movement.
It must be noted
that the use of protective grass strips is effective only if
grazing is avoided.
Crop rotation helps to preserve soil fertility.
A rotation of one
year of grain
millet, wheat, etc.) followed by three to four
years of legume pasture may be an excellent alternative to
shifting
cultivation. But
introducing crop rotation often requires a
change from traditional methods.
The new system may require new
markets, for example, as existing markets may change if
grain
crops are lost for a period of years.
Farmers may be reluctant to
adopt new cropping patterns without market incentives.
4. PHYSICAL CONTROL
OF WATER EROSION
Effective control of erosion requires either a reduction of
slope
steepness (as in terracing) or of slope length.
Both physical and
biological interventions are effective, depending on soil
character,
slope, crop cover, and land-use practice.
Frequently, a
combination of interventions gives better results than
applying
just one measure.
Vegetation Strips
Vegetation strips are contour plantings of suitably spaced
strips
of perennial grasses or shrubs on sloping lands.
The objectives
are to reduce soil and water loss, reduce slope length, hold
soils on the land, and eventually convert the barriers into
benches. Dense
vegetation strips will stop or slow runoff and
will trap moving soil particles.
It is important to give particular
attention to the layers of vegetation; dense ground covers
are more effective than vegetation with a high canopy of
trees.
Research in Taiwan showed that vegetation strips work best
on
slopes of less than 45 percent.
Spacing of strips is governed by
the distance between crop rows and is normally not more than
8
meters. If strips
are used with contour ditches, the distance between
then can be increased.
Slope is the most important factor affecting the design of
vegetation
strips. Table 1
gives approximate dimensions. For grass
barriers use fresh cuttings; plant two or three cuttings on
each
hill; plant 2 close rows to form one grass barrier.
The second
row should be planted to cover the gaps in the first row so
that
the plants in the two rows form a triangular pattern.
Table 1
Estimated Spacing Between Grass Strips
In areas where the annual rainfall is 60 to 100 cm, strip
width
and distance between strips should be increased 20 percent
and 10
percent, respectively.
With more than one meter of rainfall, increase
Width by 50 percent and Distance by 20 percent.
Slope,
Width
Distance between
percent
of strips, m
strips, m
10
5
43
20
8
38
40
13
28
60
20
20
Source: Weber &
Stoney, 1986
Horizontal Hedgerows
Horizontal hedgerows may be the simplest physical structure
for
controlling erosion on steep slopes.
To form a hedgerow, plant
single or double rows of perennial grasses or fast-growing
trees
along the contours to block runoff and catch rolling or
suspended
soil particles.
First, mark the contours and set stakes every
three to seven meters.
Plow the soil along each marked contour
into a furrow and remove the weeds before planting seeds,
seedlings,
or fresh grass cuttings.
The spacing of hedgerows depends on the slope of the
field. The
average difference in elevation between hedges should not
exceed
1.5 m, or about the distance between your eyes and your
feet. As
the slope increases this distance decreases.
Plant seedlings and
cuttings no further apart than 15 cm.
Wide spacing between trees
or grass on a contour will concentrate erosion in the gaps,
forming
rills and perhaps washing away the young plants.
Only after the seedlings are well established should they be
thinned. Remove the
weak or small seedlings leaving not more than
6 to 10 cm separating the plants.
When trees reach a height of 2
m, prune them back to about 0.5 m (knee height).
Trees are pruned
to reduce shading of crops, encourage coppicing (regrowth),
and
produce products needed by the household.
After two to seven years, terraces will develop as twigs,
stones,
and weeds are trapped on the uphill side of each hedgerow.
Sloping Agricultural Land Technology (SALT)
SALT, developed in the Philippines, has become well accepted
by
farmers to conserve soil and water.
The technology includes a
system of diversion ditches, canals, waterways, and check
dams or
soil traps on steep lands for erosion control.
Figure 1 illustrates
23p07.gif (600x600)
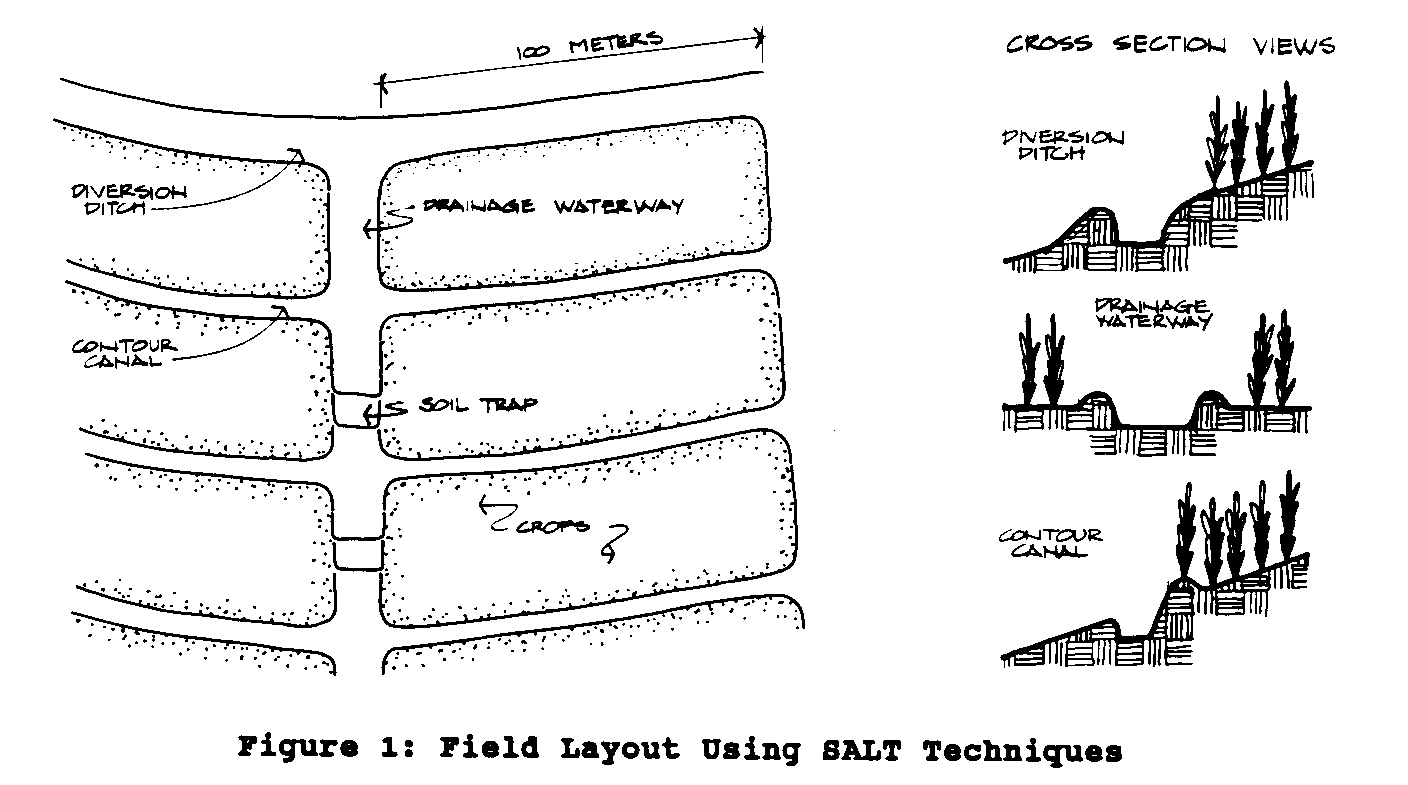
the layout and design of an erosion control system using
diversion ditches, contour canals, and drainage waterways.
Diversion ditches, the first line of defense for controlling
runoff, are designed to prevent runoff from entering the
field.
The depth and size of a diversion ditch depends on the slope
and
depth of soil. In
general diversion ditches are one meter wide
and one meter deep.
The soil from within the ditch is placed just
below the ditch where possible and planted with trees.
Contour canals control runoff within crop fields.
They are constructed
in parallel lines across the slope of the land.
A slight
gradient encourages surplus water to flow to collection
points.
On deep soils with
adequate percolation, canals are constructed
flat to hold water in the canals and increase soil-moisture
retention.
Contour canals are typically one-half meter wide and
one-half meter deep.
Wider canals can be lined with grasses.
Soil
removed in construction of canals is placed on the downslope
side
just outside the canal and planted with trees or fodder
grasses.
Drainage waterways are catchments for water collected in the
drainage ditch and contour canals.
They concentrate runoff from
the fields into constructed and managed channels.
The major objective
is to provide safe outlets for runoff and prevent soil
erosion. The
recommended dimensions of a drainage waterway are
one-half meter wide and one meter deep.
Side walls should slope
outward to reduce erosion.
Waterways are lined with grass or
stone to slow water movement and soil loss.
The distance between
waterways depends on the slope of the land and the amount of
water expected, but is usually less than 100 meters,
measured
along the diversion ditch.
When possible use natural drainage
areas; water naturally moves to these places and it will
reduce
construction costs.
Soil traps, constructed within waterways to capture
suspended
soil particles, are 1 m by 1 m pits placed every 35 meters
within
the waterway. The
trapped sediment is a source of nutrient-rich
soil to put on crop fields.
If soil conditions prohibit construction
of soil traps, check dams can be built that slow water
movement
and catch suspended soil particles.
Check dams can be constructed
from field stones, fresh branch cuttings from local
trees, sticks, or crop residue.
Branch cuttings from some trees
will sprout and form live barriers serving several purposes
by
holding subsoil with their roots, producing needed products
such
as fuelwood, and catching suspended soil particles.
Sticks and
crop residues used as the main part of check dams will decay
and
provide only short-term solutions to trapping suspended soil
particles.
The steps in laying out a SALT system are as follows:
first, mark
the location of the diversion ditch.
Then locate and mark the
contours, about 1.5 m downslope.
Remove the soil from the diversion
ditch, placing it just below the ditch and planting it with
fast growing trees or grasses.
Build the contour canals in the
same manner, with a slope of 0.5 percent to 1 percent.
Build the
drainage waterways, planting them with grasses or lining
them
with stones.
Finally, dig soil traps or build check dams.
Stone Walls
Where stones are available, stone walls can be built to
reduce
soil and water loss and gradually produce terraces.
Walls minimize
the length of slopes and removing the stones from the field
facilitates soil cultivation.
Figure 2 shows the cross-section of
23p09.gif (600x600)
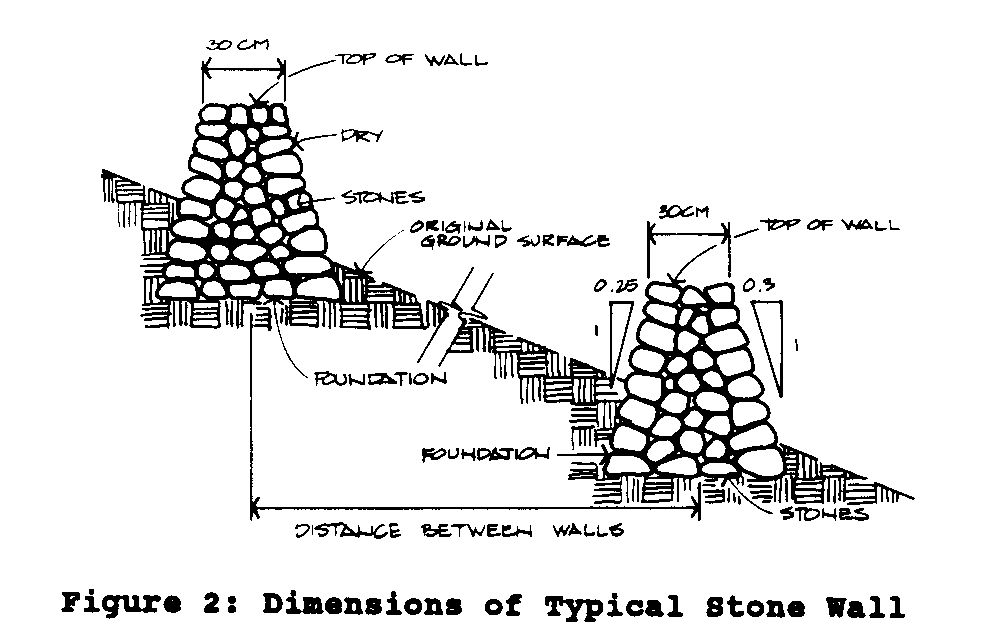
a stone wall. The
outside walls lean into the hillside, while inside
walls are almost vertical.
The top of the wall should be
about 30 cm across and the bottom about one meter.
The distance
between stone walls is determined the same way as contour
canals.
To construct a stone wall, first determine and mark the
contours
with an A-frame level.
Excavate the soil to a depth of 30 cm,
forming a flat base.
Select the largest rocks to form the foundation
and outside face. If
the wall is built after a terrace has
been formed by erosion, limit its height to 30 cm.
Terraces
Terraces are nearly-level strips built along contours.
Their main
purpose is to intercept runoff and control erosion.
Terraces
control erosion in many ways.
They segment fields into small
separate drainage areas and reduce the length of the
slope. Runoff
and its damage are reduced.
Water is conserved on the field
or moved off in a controlled manner.
Terraces reclaim eroded
lands and provide continuous protection of the reclaimed
lands.
In general, terraces are suitable on slopes up to about 50
percent.
Level terraces are best on narrow slopes; outward sloped
terraces are designed for steep land.
Unless labor is plentiful, the main constraint of
constructing
terraces is their very high labor cost.
Despite this, terraces
are the best means of soil conservation on cultivated lands.
The amount of topsoil is a factor when designing
terraces. To
ensure that the terrace can be filled, the amount of topsoil
should be not less than half the height of the riser.
The riser
should lean into the slope a little and the length should
not
exceed 100 meters.
Terrace width varies from 2 to 5 meters, depending
on several factors:
slope, depth of soil, crop spacing,
and farm operations.
To determine the Vertical Interval use the formula:
D x S VI = Vertical
Interval (m)
VI =
----- D = Width of Terrace
(m)
100 S = Slope of
Field ( percent)
Slope is calculated by:
|r
|i
rise
|s
S = ---- x 100
---------------|e
run run
Sample results are illustrated in Table 2.
The spacing in this
table may be adjusted for the type of crop and the farming
practices.
In the case of pasture grasses, with permanent cover,
ditches can be spread further apart.
To construct terraces, first survey the area and develop a
management
plan. Starting with
sites that have uniform slopes,
determine and mark the contour lines:
place the first row of
stakes at the top of the slope; walk downhill to the next
contour
line; set stakes about 3 to 7 meters apart.
Clear the land of
weeds, shrubs and trees and other obstacles.
Finally, cut and
fill starting at the bottom contour line; be sure to compact
each
filled area.
Table 2
Spacing of
Flat-Based Terraces at Various slopes
Slope,
Spacing Between Ditches
(m)
percent
Vertical Interval
Horizontal
Spacing
5
1.1
22
10
1.6
16
20
2.6
13
40
4.6
11.5
55
6.1
11.4
Source: Liau &
Wu, 1987
Figure 3 illustrates three different types of terraces.
The formula
23p11.gif (600x600)
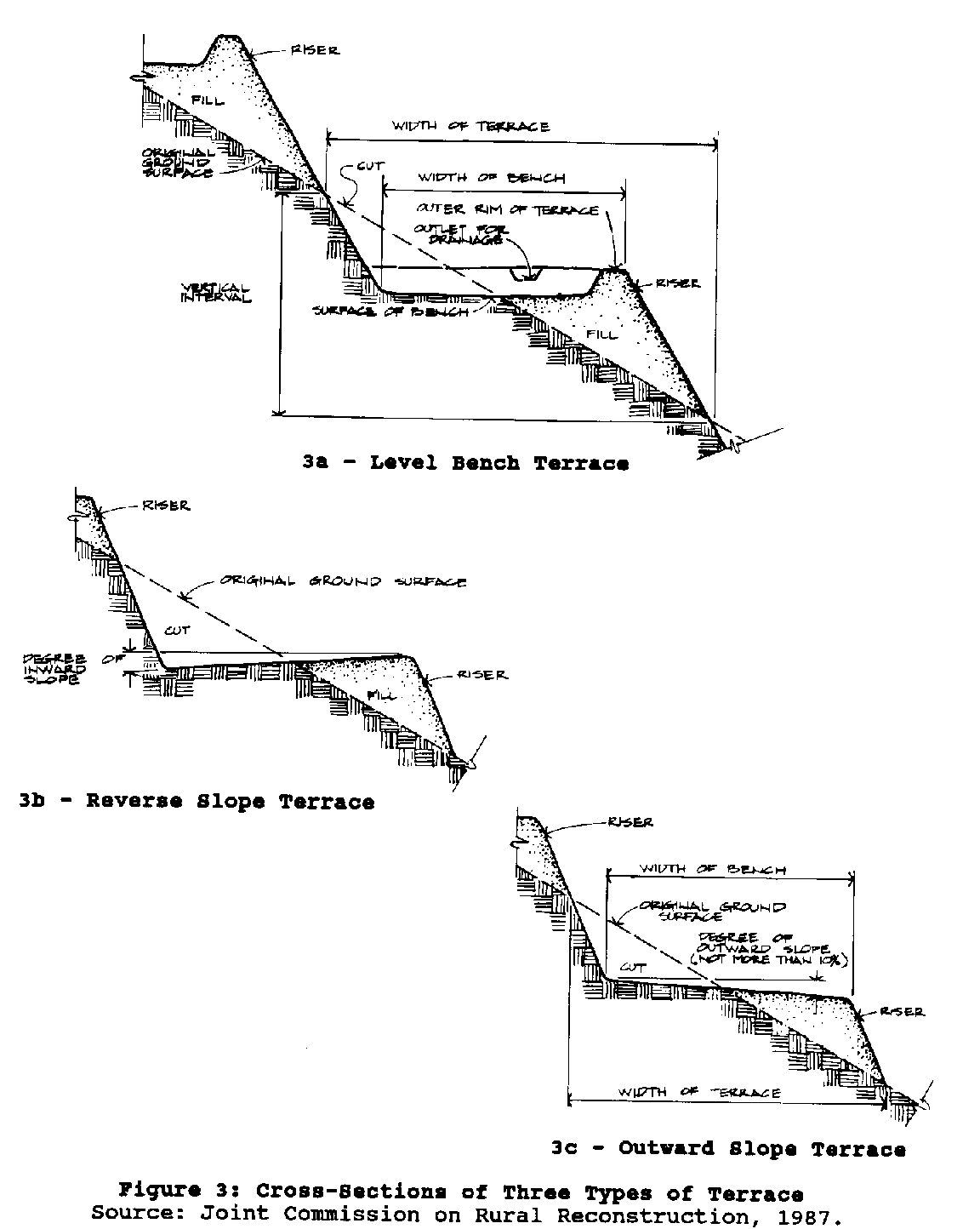
above can be used to calculate the vertical distance between
terraces for each of the three types.
The most important type of terrace for semi-arid regions is
the
flat channel terrace, sometimes known as the Zingg
conservation
bench. In Figure 4.
the vertical interval (VI), in meters, between
23p12a.gif (300x600)
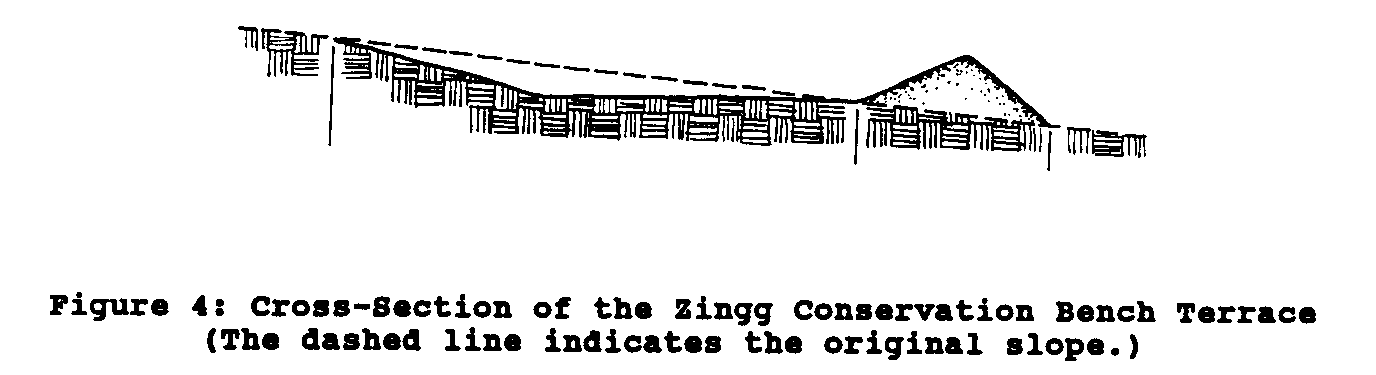
Zingg terraces is calculated by:
VI = 0.25 x
S + 0.30 VI = Vertical Interval (m)
S = slope ( percent)
An A-frame level is a simple, inexpensively built tool to
use for
mapping contours (Figure 5).
To build one, use rope or vines to
23p12b.gif (486x486)

securely fasten three
poles or bamboo pieces to
form a rigid letter "A"
2 m high and 1 m wide at
the bottom. Tie
string or
twine to the joint of two
long sticks and tie a
rock or weight to the
lower end so that it
hangs below the cross-piece
of the A.
To calibrate the A-frame level (this needs to be done only
once),
stand it on level ground and place a stake at the base of
each
leg. Mark the
crosspiece where the string passes it.
Then reverse
the leg positions of the A-frame and put another mark where
the
string passes the crosspiece.
Now put a permanent mark on the
crosspiece exactly midway between the other two marks.
In mapping
a contour, the string should always pass over this control
mark.
To use the A-frame to plot a contour canal below a diversion
ditch, walk downhill from the ditch until you can look at
the
base of the ditch without raising or lowering your
head. This is
the location of the first contour line.
Place the A-Frame on the
contour line; set a stake at the base of each leg.
Pivot the A-Frame
on one leg until the string passes over the center mark.
Set a stake at the base of the new leg position (Figure 7).
23p13b.gif (540x540)
Continue to pivot, or "wall", the A-Frame across
the slope setting
stakes at the base of each leg as the string passes the
center mark. If the
string does not pass the center mark the A-Frame
is not on the contour: adjust the placement of the forward
leg until the string is in the table right place.
Below the first contour line mark the location for the next
contour;
measure the vertical distance the same way as described
above. Continue this
process until the entire field has been
marked.
<FIGURE 6>
23p13a.gif (540x540)
Control of Gully Erosion
Gullies are surface channels that have eroded to the point
where
the land cannot be smoothed by normal tillage
practices. They
form when large amounts of water accumulate and concentrate
erosion
in rills that deepen and form V-gullies or U-gullies, named
for the shapes of their cross-sections (Figure 7).
23p13b.gif (600x600)
Check dams, made of locally available materials such as
rocks,
stones, stakes, freshly cut branches, sacks of soil, can be
built
to shorten gully length and reduce runoff velocity.
Areas above
check dams fill with sediment and form terraces.
The base of each
dam should be level with the top of the next downhill check
dam.
The top of each dam should be concave to allow excess water
to
flow over its center and should extend past the sidewalls of
the
gully. Branches cut
from some trees will sprout and form live
barriers serving several purposes by holding subsoil with
their
roots, producing needed products such as fuelwood, and
catching
suspended soil particles.
Impermeable check dams prevent water
and sediment from moving downslope.
V-Gullies. V-shaped
gullies form with downward cutting of the
center of the channel.
The gradient of the channel center is
greater than the slope of the field.
Typically, V-gullies deepen
downslope and grow in length upslope.
Water flows through V-gullies
in small amounts but with high velocities.
V-gullies should be eliminated.
If shallow, they can be filled
with new soil.
Immediate control measures are needed to assure
that they do not re-appear.
Other methods to control V-gully
erosion include contour cultivation and strip cropping.
A diversion
ditch should be constructed around the top of the gully.
Protect the outlets of diversion ditches from erosion.
Construct
permeable check dams within V-gullies to slow down the flow
of
water and catch sediment (Figure 8).
The distance between check
23p14.gif (486x486)

dams depends on slope and amount of runoff; make dams closer
together on steep slopes.
U-Gullies. The flat
bottoms of U-shaped gullies have slopes parallel
to the slope of the land.
Water flow is greater, but the
velocity is much less than in V-gullies.
Control starts at the
points where they grow, the head (length) and sides (width).
First, raise the bottom of the channel by constructing a
series
of permanent, impermeable check dams (Figure 9).
Eventually, the
23p15.gif (486x486)
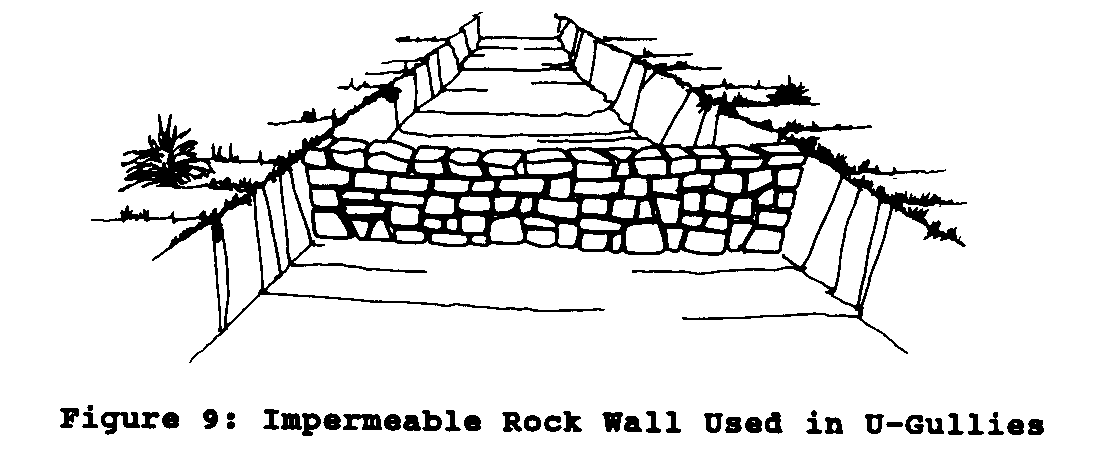
area uphill of each check dam fills with sediment, raising
the
bottom of the U-gully.
Reshape gully walls so that for every
meter of rise one meter of horizontal distance is
covered. Finally,
stabilize the channel by planting grasses, vines, or shrubs.
5. CONTROL OF WIND
EROSION
Strong wind detaches soil particles from the surface,
transports
them, and deposits them downwind.
Two danger signs of possibly
harmful wind erosion are sand buildup on the downwind sides
of
obstacles and sediment ripples in fields.
Even in a short time,
wind can blow away enough soil to greatly reduce soil
fertility
and crop yields.
Wind may expose recently planted seed and prevent
germination. The
abrasive power of soil particles suspended
in the wind can permanently damage small plants.
Loose, dry, and finely granulated soil particles are blown
away
more easily than heavier textured soils.
Wind erosion is favored
by sandy soils, smooth surfaces, sparse vegetation, open
expanses
of land, and strong or turbulent winds.
Accordingly, control
measures include increasing soil stability and surface
roughness.
Tillage can compress soils and smooth the surface, and
should be
limited to the adequate preparation of seed beds and the
control
of weeds.
Conservation cultivation, particularly minimum tillage,
is a practical method to stabilize soils.
Physical barriers should be perpendicular to the wind
direction.
A windbreak is a dense barrier of perennial tree crops and
shrubs
specifically designed to reduce wind speed for the benefit
of
annual crops (Figure 10).
Well planted and well grown windbreaks
23p16a.gif (540x540)
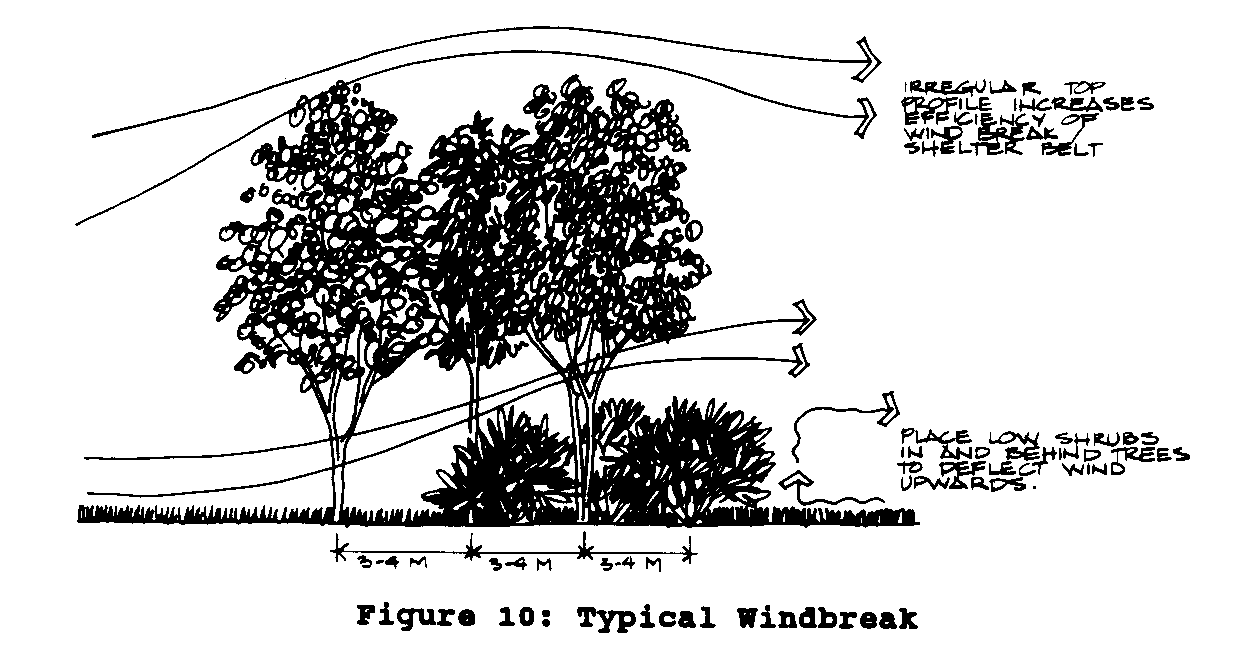
can reduce wind velocity by as much as 70 percent to 80
percent
near the barrier.
Moreover, a windbreak can modify air temperature
within the protected areas and conserve soil moisture by
reducing evapotransporation.
The relative humidity within the
canopy on the downwind side increases.
Another important side
effect, especially if already-limited cropland must be taken
out
of production to plant the windbreak, is the fruit, fuel,
nuts,
or other produce of the trees.
The effect of a windbreak is proportional to its
height. In general
the reduction of wind velocity past the windbreak weakens
and becomes negligible at a distance of 30 to 40 times the
its
height. The density
of windbreaks also affects the decrease in
wind velocity (Fig 11).
A dense windbreak reduces velocity sharply
23p16b.gif (540x540)
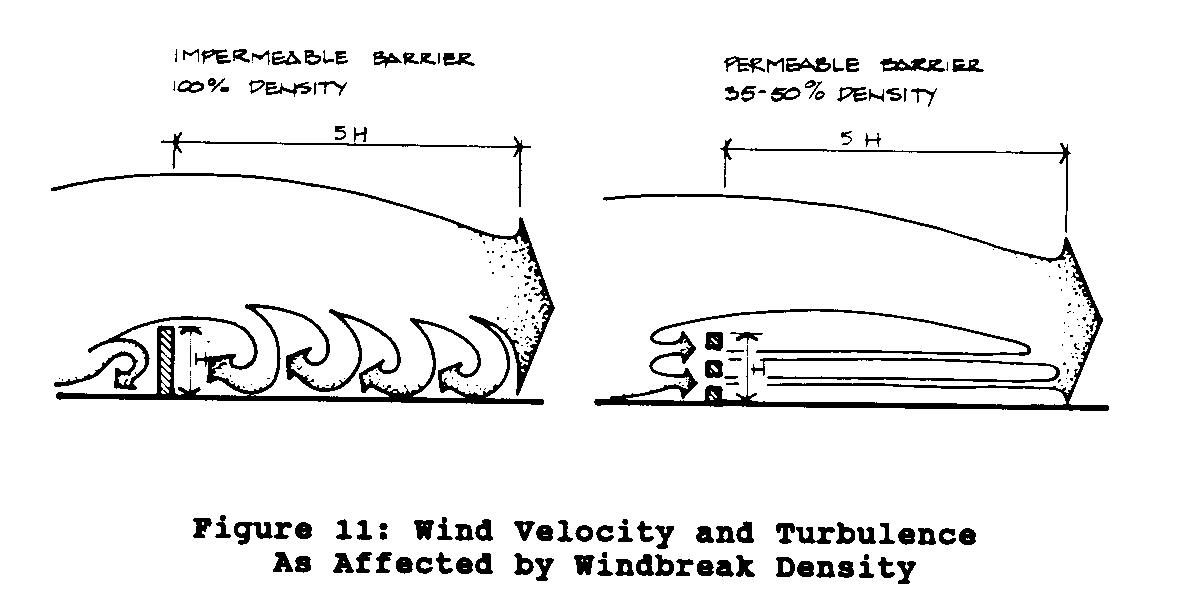
and quickly. A
windbreak that is too dense causes the wind
velocity to recover in a shorter distance, thus reducing the
length of the protected area.
The most effective density is between
35 percent and 50 percent.
The distance between windbreaks (Figure 12) is critical, but
23p17a.gif (540x540)
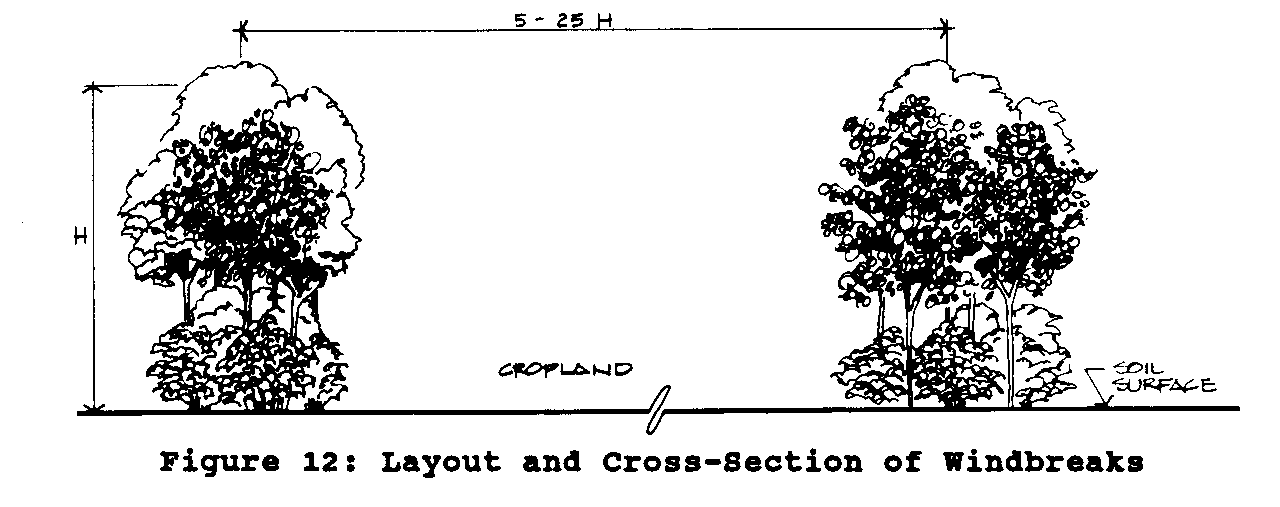
varies with crops and soil stability.
The best distance between
barriers shielding forage crops is 10 to 14 times the
height. In
areas with highly erodible soils, strong winds, or sensitive
crops (fruit or vegetables) the distance between windbreaks
should be 5 to 10 times the height of the barrier.
For moderately
responsive crops (wheat, rye, oats, etc.) the distance is
extended
to 15 to 25 times the height of barriers.
Windbreaks should extend the total length of the field and
run
perpendicular to the wind direction.
Gaps or breaks will accelerate
wind through them and increase erosion; allowance for
necessary
pathways or stock crossings should be made on the diagonal
(Figure 13).
Windbreaks not perpendicular to wind direction will
23p17b.gif (540x540)
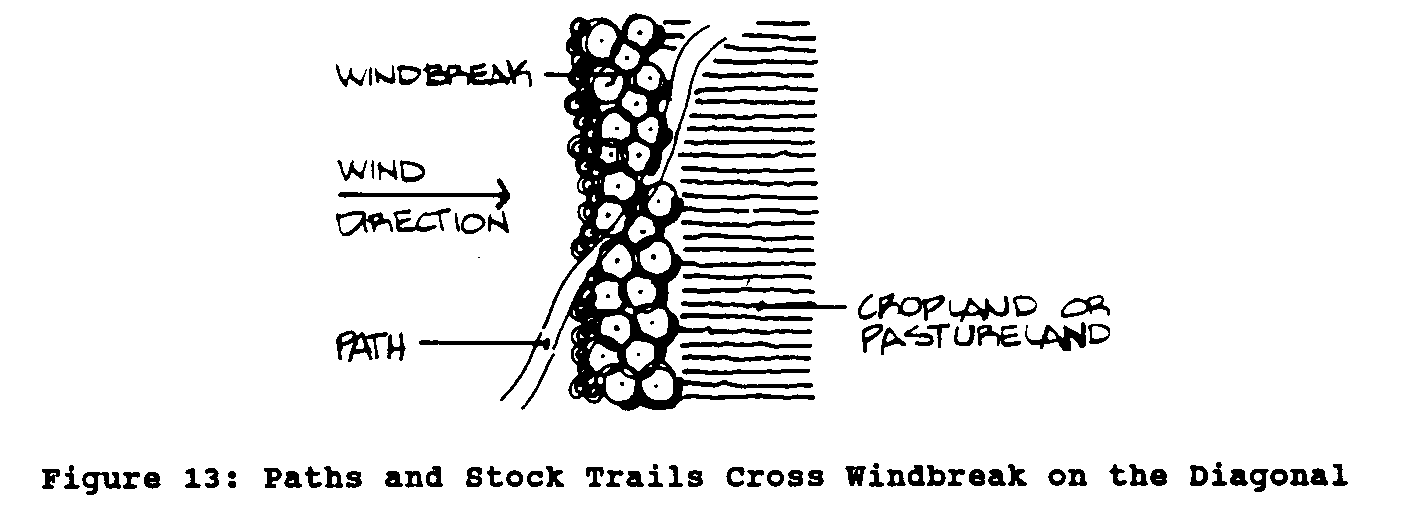
channel wind along the barriers.
The best shape for windbreaks is
created by multiple rows of trees, but this takes more land
out
of crop production.
Local tree species that send deep tap roots
and develop narrow crowns are best.
To keep windbreaks viable it is essential to maintain the
vigor
and growth of the trees by thinning and cutting when
necessary.
6. PLANNING FOR
EROSION CONTROL
Individually, the control measures discussed above reduce
runoff
and slow erosion under specific conditions.
However, maximal
control of erosion is achieved through planned activities
that
use a variety of control measures.
Effective planning involves
selecting and developing the best course of action to reduce
or
halt the movement of soil from crop fields while maintaining
farm
productivity.
It is essential first to collect all available data about
the
land. Critical
information for land use planning includes soil
depth, soil type, drainage characteristics, and slope of the
land. A field survey
should assess the target area for the severity
of erosion; consider the extent of sheet erosion, the space
between rills, and the type and spacing of gullies; and
determine
the texture class of the soil.
The field survey also should consider
the abundance of stones; the consistency, structure, and
stability of the surface; and soil reaction, salinity, and
drainage.
Frequency, duration, and intensity of rain and wind should
also be noted.
In addition, the survey should look at tillage and animal
husbandry
practices in use, and the resources farmers have available
to make necessary changes.
In this regard, it is important to
engage the farmers' interest and participation by assuring
that
they are intimately involved in the survey and planning
process.
The practices selected to control erosion should be based on
a
combination of principles.
First, the practices should maintain
soil infiltration rates at high levels to reduce runoff to
negligible
amounts. Examples
are mulching and vegetation cover.
Second,
they should safely dispose of runoff from the field.
Such
physical structures as hedgerows, contour canals, stone walls,
and terraces are used for this.
Finally, practices must be within
the means of farmers to implement and maintain, or they will
not
be continued more than a season or two.
SOURCES
OF INFORMATION (FURTHER READINGS)
Addresses are in the United States unless otherwise stated.
El-Swaify, S.A., Moldenhauer, W.C. and Lo, A. (eds).
Soil Erosion
and Conservation.
Proceedings of an international conference held
in Honolulu, Hawaii, January 16-22, 1983.
Ankeny, Iowa:
Soil
Conservation Society of America, 1985.
United Nations. Food
and Agriculture Organization.
Guidelines for
Watershed Management.
FAO Conservation Guide No. 1. Rome (Italy):
FAO, 1977.
Finkel, H.J., Finkel, M., and Naveh, Z. (eds.)
Semi-arid Soil &
Water Conservation.
Boca Raton, Florida: CRC Press,
1986.
Follet, R.F. and Stewarts, B.A. (eds.) Soil Erosion and
Productivity.
Madison, Wisconsin:
American Society of Agronomy,
Crop Science Society of America, and Soil Science Society of
America, 1985.
Greenland, D.J. and Lal, R. Soil Conservation and Management
in
the Humid Tropics.
New York: Wiley, 1977.
International Institute of Rural Reconstruction.
Agroforestry
Technology Information Kit, the output of a workshop (texts
and
illustrations) November 4-13, 1989.
New York:
IIRR (Room 1270,
475 Riverside Drive, New York, New York 10115), 1990.
Joint commission on Rural Reconstruction.
Soil Conservation
Handbook, rev. ed. Taipei (Taiwan):
Food and Fertilizer Technology
Center, 1987.
Liao, Mein-Chun, and Wu, Huei-Long.
Soil Conservation on Steep
Land in Taiwan.
Taipei, (Taiwan): The Chinese
Soil and Water
Conservation Society, 1987.
MacDicken, K.G. and Vergara, N.T.
Agroforestry:
Classification
and Management. New
York: Wiley, 1989.
Moldenhauer, W.C. and Hudson, N.W. (eds).
Conservation Farming on
Steep Lands.
Proceedings of an international workshop, San Juan,
Puerto Rico, 22-27 March 1987.
Ankey, Iowa: Soil and
Water Conservation
Society, 1988.
O'Loughlin, C.L. and Pearce, A.J. Symposium on Effects of
Forest
Land Use on Erosion and Slope Stability.
Proceedings of a symposium
held in Honolulu, Hawaii, May 1984.
Honolulu:
East-West
Center, 1984.
Pearce, A.J. and Hamilton, L.S. Water and Soil Conservation
Guidelines for Land Use Planning.
Report of a seminar.
Honolulu,
Hawaii: East-West
Center, 1986.
Schiechtl, H.M., and Michaelson, T. FAO Watershed Management
Field Manual; Vegetative and Soil Treatment Measures.
FAO Conservation
Guide 13/1. Rome
(Italy): Food and Agriculture organization
of the United Nations, 1985.
Weber, F.R. with Stoney, C. Reforestation in Arid
Lands. Arlington,
Virginia: Volunteers
in Technical Assistance, 1986.
========================================
========================================